Cancer Drug Targets
Related Symbol Search List
- ABL1
- ADORA2A
- AKT1
- ANGPT1
- Angpt2
- ANXA3
- AR
- Aurora A
- AURKB
- Bcl-2
- BRAF
- BTK
- BTLA
- BTN2A1
- CA9
- CCR4
- CD109
- CD160
- CD19
- CD200
- CD200R
- CD22
- CD226
- CD24
- CD244
- CD27
- CD274
- CD276
- CD28
- CD33
- CD36
- CD37
- CD38
- CD40
- CD40 Ligand
- CD47
- CD48
- CD52
- CD70
- CD79B
- CD80
- CD86
- CD96
- CDK4
- CDK9
- CEACAM1
- CEACAM5
- Chk1
- CHEK2
- CSF1R
- Csf2
- Ctla4
- CXCR2
- CXCR4
- DDR2
- DLL3
- DLL4
- EGFR
- ENG
- Her2
- ERBB2
- HER3
- ERBB3
- ErbB4
- FAP
- CD16a
- FGF2
- FGFR1
- FGFR2
- FGFR3
- FGFR4
- FLT1
- FLT3
- FLT4
- FOLH1
- GPA33
- GPC3
- HAVCR2
- HDAC1
- HDAC2
- HDAC3
- HDAC4
- HDAC5
- HDAC8
- HDAC9
- HGF
- HHLA2
- HIF1A
- HSP90AA1
- HSP27
- HSPB1
- ICOS
- Icosl
- IDO1
- IGF1R
- IL-13
- IL2RA
- Il6
- JAK1
- JAK2
- JAK3
- Kdr
- KIT
- KLRC1
- KRAS
- LAG3
- LGALS9
- MEK1
- MAP2K2
- ERK2
- ERK1
- MAPK3
- MDM2
- MET
- MMP1
- MMP10
- MMP11
- MMP13
- MMP-2
- MMP7
- MMP-9
- MS4A1
- MTOR
- MUC1
- MYC
- NRAS
- TrkA
- TrkB
- TrkC
- PARP1
- KIAA0101
- PDCD1
- PD-L2
- PDGFRA
- PDGFRB
- PIK3CA
- PIK3CB
- PIK3CD
- PIM1
- PIM3
- PSENEN
- PTEN
- PTGS2
- PTK2
- PVR
- RAF1
- RET
- S100A4
- SIRPA
- SLAMF7
- SMO
- Src
- STAT3
- STEAP1
- TROP2
- TDO2
- TIE2
- Tgfb1
- TIGIT
- TLR3
- TLR8
- CD28H
- TMIGD2
- TNFa
- TNFRSF10A
- Tnfrsf10b
- HVEM
- Tnfrsf18
- TNFRSF4
- TNFRSF8
- TNFRSF9
- TNFSF9
- TNFSF10
- RANKL
- LIGHT
- TNFSF18
- Tnfsf4
- TNFSF9
- p53
- VEGFA
- VEGFA
- VTCN1
Immunology Background
Background
Cancer remains one of the most challenging diseases to treat, characterized by uncontrolled cell growth and proliferation. Despite advances in medical research, the heterogeneity and adaptability of cancer cells pose significant obstacles to effective treatment. One promising approach in the fight against cancer is the development of targeted therapies. Unlike conventional chemotherapy, which indiscriminately attacks rapidly dividing cells, targeted therapies aim to disrupt specific molecular mechanisms crucial for cancer cell survival and proliferation.
Mechanisms of Action
Targeted cancer therapies operate by interfering with specific molecules involved in the growth, progression, and spread of cancer. These therapies are designed based on the understanding of molecular pathways and genetic alterations unique to cancer cells. Key mechanisms include:
Inhibition of Cell Signaling Pathways
Cancer cells often rely on aberrant signaling pathways for growth and survival. Targeted therapies can inhibit these pathways, leading to cell death. For example, the inhibition of the epidermal growth factor receptor (EGFR) and the human epidermal growth factor receptor 2 (HER2) pathways can suppress tumor growth.
Induction of Apoptosis
Many cancer cells evade programmed cell death (apoptosis). Targeted therapies can reactivate apoptotic pathways, causing cancer cells to self-destruct. Drugs such as BCL-2 inhibitors work by promoting apoptosis.
Angiogenesis Inhibition
Tumors require blood vessels to supply nutrients and oxygen. Angiogenesis inhibitors, such as bevacizumab, block the growth of new blood vessels, starving the tumor.
Epigenetic Modulation
Some targeted therapies modify the expression of genes involved in cancer progression without altering the DNA sequence. Histone deacetylase inhibitors and DNA methyltransferase inhibitors are examples that affect gene expression through epigenetic changes.
Types of Targets
1. Kinases as targets
Kinase inhibitors are the class of anticancer drugs that interact directly with the active site of the target enzyme and inhibit kinase function. Several small molecule kinase inhibitors have been investigated as anti-tumor agents and approximately 80 inhibitors are in advanced clinical trials. Protein kinases are involved in most signal transduction in cells, reversibly phosphorylate proteins in post-translational modifications, and regulate most cellular activities. Some of the important kinases and their role in anticancer drug development are described below:
1.1 Tyrosine Kinases
TKs are enzymes that transfer a phosphate group from ATP to tyrosine residues of specific proteins, a critical process in signaling pathways that regulate various cellular functions, including growth, differentiation, metabolism and apoptosis. Dysregulation of TKs is a common feature of many cancers, making them important targets for anticancer drug development. Tyrosine kinases can be broadly classified into receptor tyrosine kinases (RTKs) and non-receptor tyrosine kinases (NRTKs).
Receptor Tyrosine Kinases (RTKs) are transmembrane proteins that, upon binding with specific ligands (such as growth factors), undergo dimerization and autophosphorylation, activating intracellular signaling pathways that control cell proliferation, survival, and migration. Dysregulation of RTKs, through overexpression or mutation, leads to aberrant signaling that promotes oncogenesis.
Non-Receptor Tyrosine Kinases (NRTKs) function in the cytoplasm and nucleus, playing essential roles in intracellular signaling pathways that regulate the cell cycle, migration, apoptosis, and differentiation. Abnormal activity of NRTKs due to mutations or chromosomal translocations has been implicated in several cancers.
Key RTKs and targeted drugs are summarized in Table 1 below:
Tab. 1: Tyrosine kinase as cancer drug targets (Lee et al., 2011).
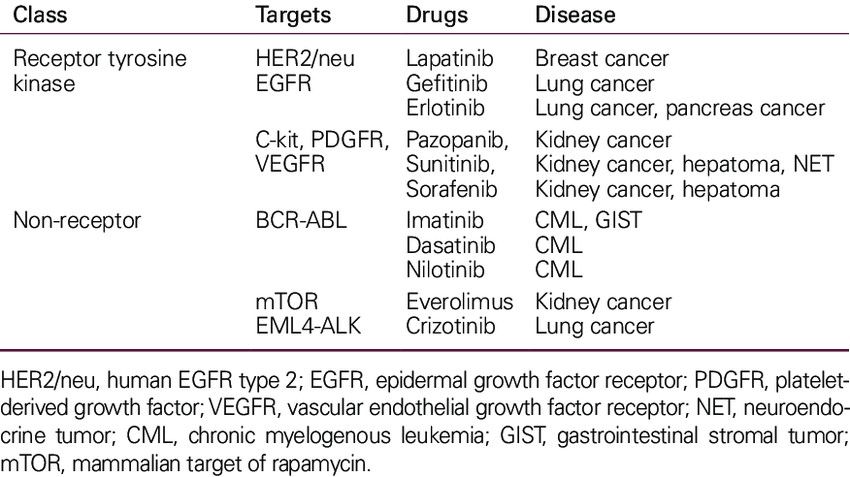
1.2 Cyclin Dependent Kinases
Cyclin-dependent kinases (CDKs) are a family of serine/threonine kinases that play a critical role in the regulation of the cell cycle. CDKs form complexes with cyclins, and these complexes are essential for the progression of cells through various phases of the cell cycle. Dysregulation of CDKs, often due to overexpression of cyclins or loss of CDK inhibitors, leads to uncontrolled cell division, a hallmark of cancer. Genetic and epigenetic alterations of CDK pathway components in human cancers are summarized in Table 2. The corresponding targeted drugs are shown in Figure 1.
Tab. 2: Genetic and epigenetic alterations of Cdk pathway components in human cancer (Graf et al., 2010; Ortega et al., 2002; Weinberg, 2007).
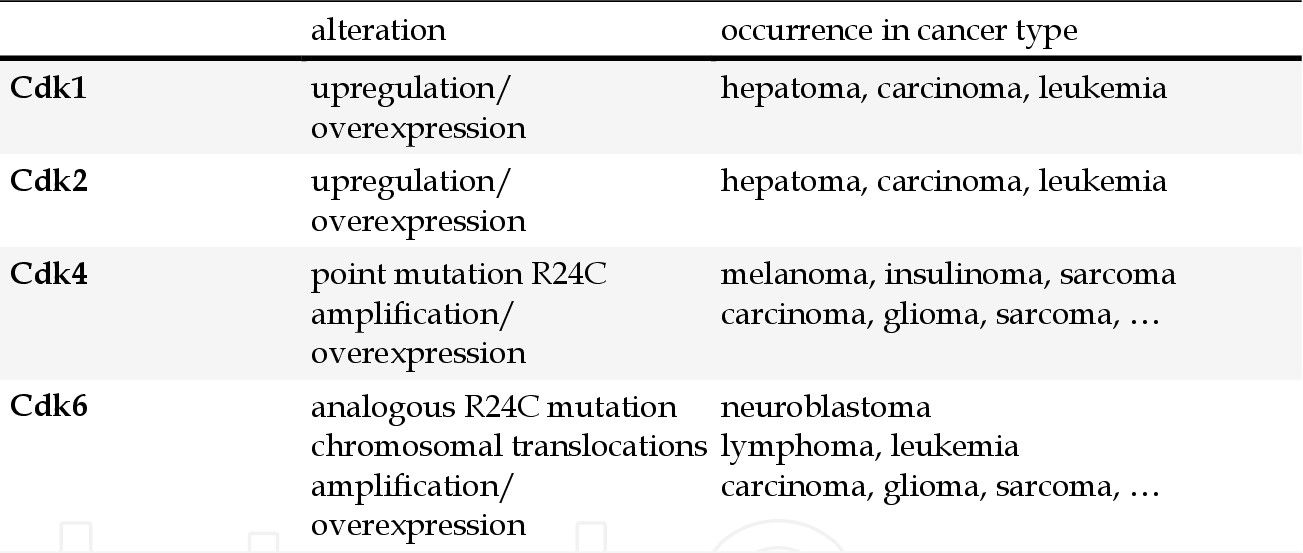
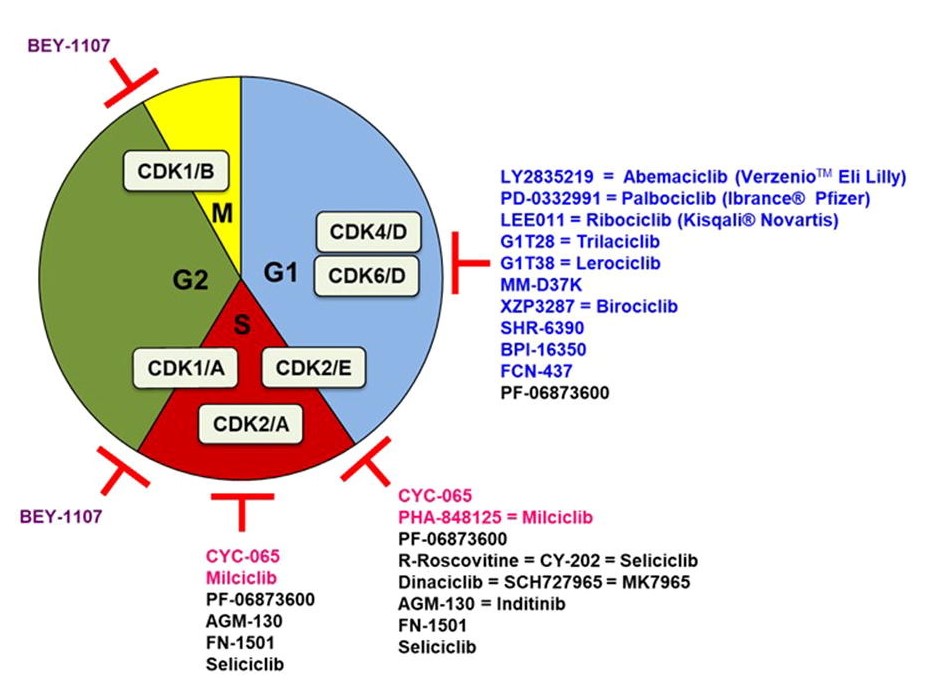
2. Tubulin/microtubule as a target
Microtubules are dynamic structures composed of α- and β-tubulin heterodimers that play essential roles in cell shape, intracellular transport, and mitosis. The dynamic instability of microtubules, characterized by phases of growth and shrinkage, is critical for proper cell division. Disruption of microtubule dynamics can inhibit mitosis, leading to cell cycle arrest and apoptosis. This makes tubulin/microtubules an important target in cancer therapy, particularly for rapidly dividing tumor cells.
2.1 Microtubule Stabilizing Agents
MSAs bind to and stabilize microtubules, preventing them from depolymerizing. This leads to the formation of overly stable microtubules that cannot undergo the dynamics necessary for cell division. The resulting mitotic arrest triggers apoptosis in cancer cells. Examples include paclitaxel (Taxol), widely used in the treatment of breast, ovarian and lung cancer; docetaxel (Taxotere), used in breast, prostate and non-small cell lung cancer (NSCLC); and epothilones (e.g., ixabepilone) for paclitaxel-resistant cancers, including metastatic breast cancer.
2.2 Microtubule Destabilizing Agents
MDAs bind to tubulin and inhibit microtubule polymerization, leading to microtubule depolymerization. This disruption of microtubule dynamics interferes with spindle assembly during mitosis, leading to cell cycle arrest and apoptosis. Examples include vinca alkaloids (e.g., vincristine, vinblastine, vinorelbine) used in the treatment of Hodgkin's lymphoma, non-Hodgkin's lymphoma, breast cancer, etc.; eribulin (Halaven) used in the treatment of metastatic breast cancer and liposarcoma.
3. Vascular targeting agents
Vascular targeting agents (VTAs) represent a unique class of cancer therapeutics designed to disrupt the tumor vasculature. Tumors require a constant supply of oxygen and nutrients to grow and metastasize, which is facilitated by the formation of new blood vessels through a process called angiogenesis. VTAs work by either inhibiting the formation of new blood vessels (anti-angiogenic agents) or directly damaging the existing tumor vasculature (vascular disrupting agents).
3.1 Anti-Angiogenic Agents
Anti-angiogenic agents inhibit the growth of new blood vessels necessary for tumor survival and growth. They target key molecules involved in the angiogenesis process, primarily the vascular endothelial growth factor (VEGF) and its receptors (VEGFRs). By blocking these pathways, anti-angiogenic agents effectively starve the tumor of nutrients and oxygen. Key targets are VEGF/VEGFR pathway and other pathways includes platelet-derived growth factor (PDGF) and fibroblast growth factor (FGF) pathways.
3.2 Vascular Disrupting Agents (VDAs)
VDAs target the established tumor vasculature, causing rapid and selective damage to the blood vessels within the tumors. This results in acute disruption of blood flow, leading to extensive tumor cell death due to ischemia. Unlike anti-angiogenic agents, VDAs do not prevent the formation of new blood vessels but rather disrupt existing ones. Key targets are microtubules, VEGFR and integrins.
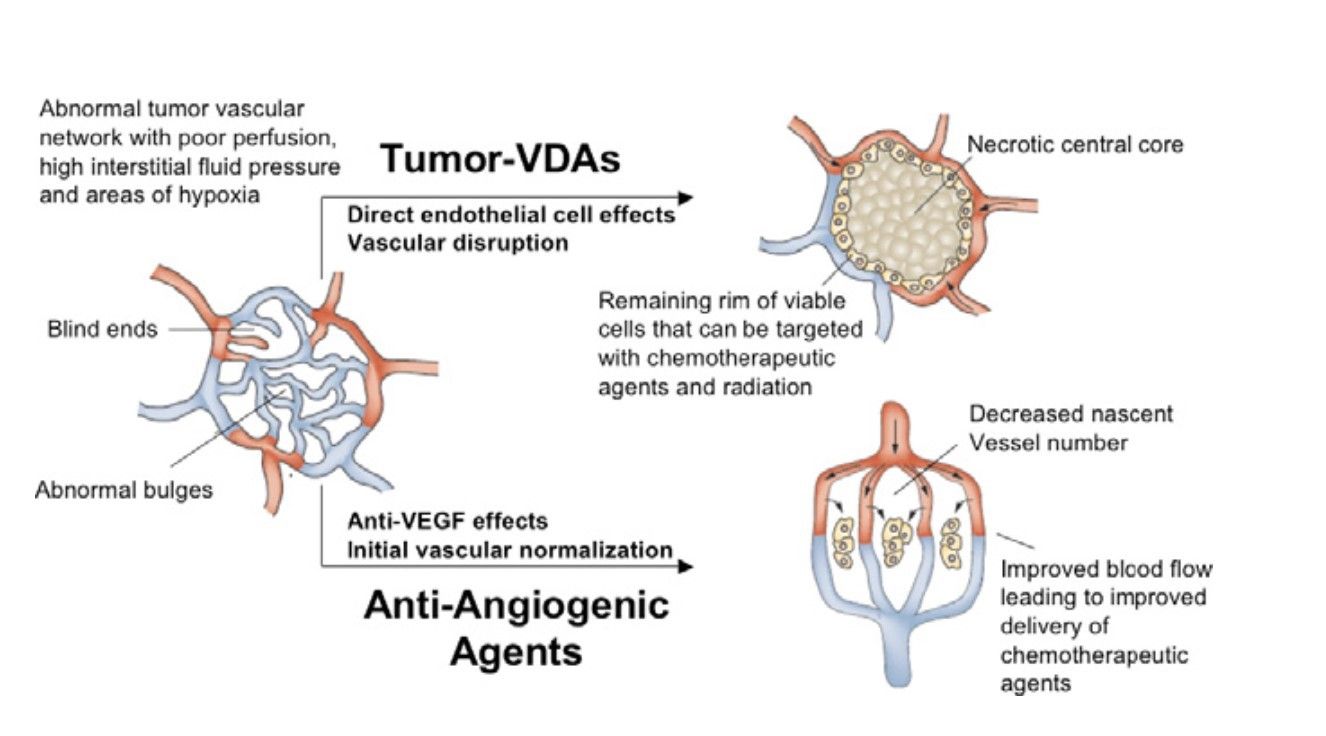
4. Cancer stem cells as a target
Cancer stem cells (CSCs) are a small subpopulation of cancer cells with the ability to self-renew, differentiate, and initiate tumor growth. These cells are believed to play crucial roles in tumor progression, metastasis, and recurrence. Targeting CSCs holds promise for more effective cancer therapies. Key signaling pathways involved in maintaining the properties of CSCs include the Notch, Hedgehog, Wnt, and NF-kB pathways.
5. Multi-targeting anti-cancer agents
Multi-targeting anti-cancer agents are therapeutic compounds designed to inhibit multiple molecular targets simultaneously. Unlike traditional therapies, which often target a single pathway or molecule, multi-targeting agents aim to disrupt multiple key pathways involved in cancer progression, survival and resistance. This approach can increase therapeutic efficacy, reduce the likelihood of drug resistance and improve clinical outcomes for patients.
Multi-targeting anti-cancer agents work by simultaneously inhibiting different components of the molecular pathways that drive tumor growth and survival. These agents can target multiple receptors, kinases and other proteins involved in cell proliferation, apoptosis, angiogenesis and metastasis. Key mechanisms include simultaneous inhibition of multiple kinases, interference with multiple signaling pathways, combination of anti-angiogenic and anti-proliferative effects, and dual targeting of cancer cells and the tumor microenvironment. For example, sorafenib (Nexavar) inhibits multiple kinases, including Raf-1, B-Raf, VEGFR-2, VEGFR-3, PDGFR-β, c-KIT and FLT-3. The drug is approved for the treatment of hepatocellular carcinoma, renal cell carcinoma and thyroid cancer.
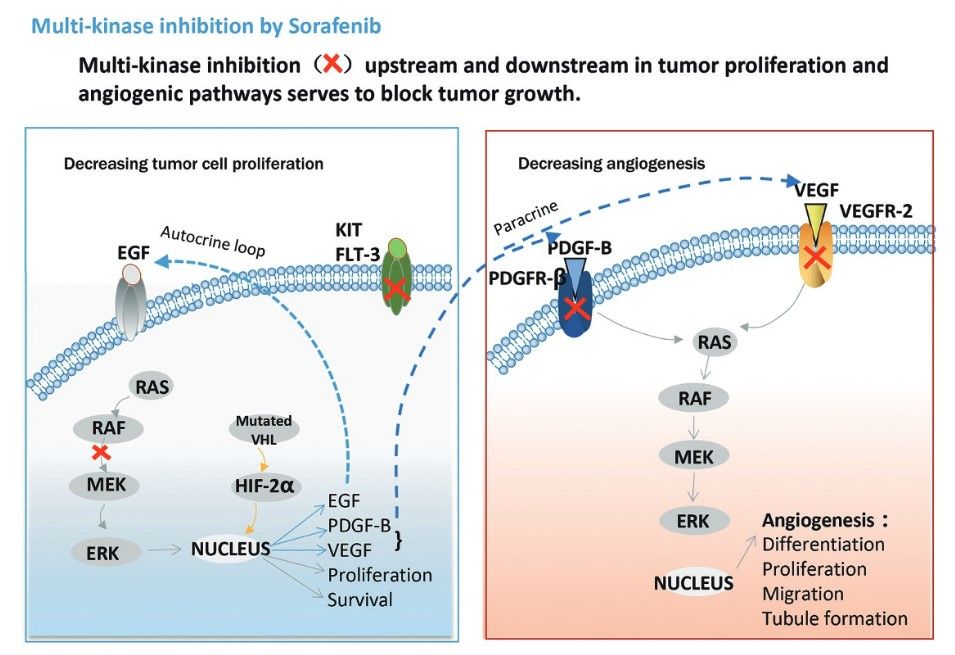
The development of targeted therapies has revolutionized cancer treatment, offering more personalized and effective options than traditional chemotherapy. By understanding and exploiting the unique molecular characteristics of cancer cells, these therapies offer new hope for patients with previously untreatable or refractory cancers. However, challenges remain, including drug resistance and side effects. Ongoing research is essential to develop new targets and improve existing therapies, with the goal of a future in which cancer can be managed as a chronic, if not curable, disease.
Case Study
Case 1: Shi, Junwei.; et al. Discovery of cancer drug targets by CRISPR-Cas9 screening of protein domains. Nature Biotechnology, vol. 33, no. 6, June 2015, pp. 661–67.
In addition to its widespread use to generate gene-specific knockouts in a variety of biological systems, CRISPR-Cas9 genome editing technology holds great promise for the discovery of therapeutic targets in cancer and other diseases. Current screening strategies target CRISPR-Cas9-induced mutations in the 5' exons of candidate genes, but this approach often produces in-frame variants that retain functionality, which can obscure even strong genetic dependencies. In this study, the researchers overcome this limitation by targeting CRISPR-Cas9 mutagenesis to exons encoding functional protein domains. This generates a higher proportion of null mutations and significantly increases the power of negative selection. Using this advanced approach, a screen of 192 chromatin regulatory domains in murine acute myeloid leukemia cells identifies six known drug targets and 19 additional interdependencies.
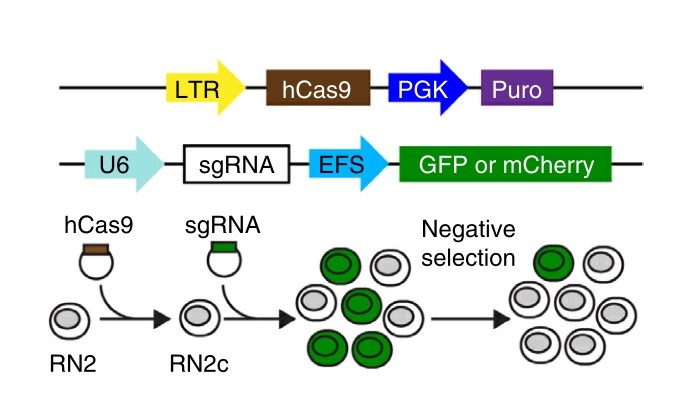
References
- Graf, Franziska, et al. "Cyclin-Dependent Kinases (Cdk) as Targets for Cancer Therapy and Imaging." Advances in Cancer Therapy, edited by Hala Gali-Muhtasib, InTech, 2011. DOI.org (Crossref), https://doi.org/10.5772/24852.
- Heath, Victoria L., and Roy Bicknell. "Anticancer Strategies Involving the Vasculature." Nature Reviews. Clinical Oncology, vol. 6, no. 7, July 2009, pp. 395–404. PubMed, https://doi.org/10.1038/nrclinonc.2009.52.
- Kumar, Bhupinder, et al. "Promising Targets in Anti-Cancer Drug Development: Recent Updates." Current Medicinal Chemistry, vol. 24, no. 42, 2017, pp. 4729–52. PubMed, https://doi.org/10.2174/0929867324666170331123648.
- Lee, Namsu, et al. "Oral chemotherapeutic agents in current use." Journal of the Korean Medical Association, vol. 54, no. 11, 2011, p. 1191. DOI.org (Crossref), https://doi.org/10.5124/jkma.2011.54.11.1191.
- Sánchez-Martínez, Concepción, et al. "Cyclin Dependent Kinase (CDK) Inhibitors as Anticancer Drugs: Recent Advances (2015–2019)." Bioorganic & Medicinal Chemistry Letters, vol. 29, no. 20, Oct. 2019, p. 126637. ScienceDirect, https://doi.org/10.1016/j.bmcl.2019.126637.
- Shi, Junwei, et al. "Discovery of Cancer Drug Targets by CRISPR-Cas9 Screening of Protein Domains." Nature Biotechnology, vol. 33, no. 6, June 2015, pp. 661–67. www.nature.com, https://doi.org/10.1038/nbt.3235.
- Zhu, Yan-jing, et al. "New Knowledge of the Mechanisms of Sorafenib Resistance in Liver Cancer." Acta Pharmacologica Sinica, vol. 38, no. 5, May 2017, pp. 614–22. www.nature.com, https://doi.org/10.1038/aps.2017.5.