Excretory System Development
Creative BioMart Excretory System Development Product List
Immunology Background
Background
The excretory system is essential for maintaining homeostasis by removing waste products and excess substances from the body. This system consists of a network of organs and tissues that filter blood, regulate fluid balance, and eliminate waste products in the form of urine. The development of the excretory system is a complex process that involves the coordinated formation of the kidneys, ureters, bladder, and urethra, regulated by specific genetic and molecular signals.
Overview of the Excretory System
The excretory system primarily includes the kidneys, ureters, bladder, and urethra.
- Kidneys: The primary organs responsible for filtering blood, removing wastes, and producing urine. Nephrons are the functional units responsible for filtration. The filtrate then passes through the renal tubules, where essential substances such as glucose, salts, and water are reabsorbed into the bloodstream. The remaining waste products and water form urine, which is collected in the renal pelvis and directed to the ureters. The kidneys also play an important role in regulating blood pressure, electrolyte balance, and red blood cell production. They do this by secreting hormones such as renin, which controls blood pressure, and erythropoietin, which stimulates red blood cell production in the bone marrow.
- Ureters: Muscular tubes that transport urine from the kidneys to the bladder. The walls of the ureters are lined with smooth muscle fibers that contract rhythmically in a process known as peristalsis, propelling urine from the renal pelvis in the kidneys down to the bladder. The ureters have a crucial role in ensuring that urine flows in one direction, preventing any backflow that could lead to infections or damage to the kidneys.
- Bladder: A hollow organ that stores urine until it is ready to be expelled from the body. It is highly elastic and can expand significantly when filled with urine. The bladder has two main functions: storage and controlled release of urine. As the bladder fills, stretch receptors in its walls signal the brain when it has reached a certain capacity, triggering the urge to urinate.
- Urethra: The tube that carries urine from the bladder out of the body during urination. The urethra is lined with mucous membranes and surrounded by smooth muscle that helps control the flow of urine. The urethra plays a key role in the mechanism of urinary continence.
These components of the excretory system work together seamlessly to ensure the efficient removal of waste products and the maintenance of homeostasis, which is critical for the body's overall health and function.
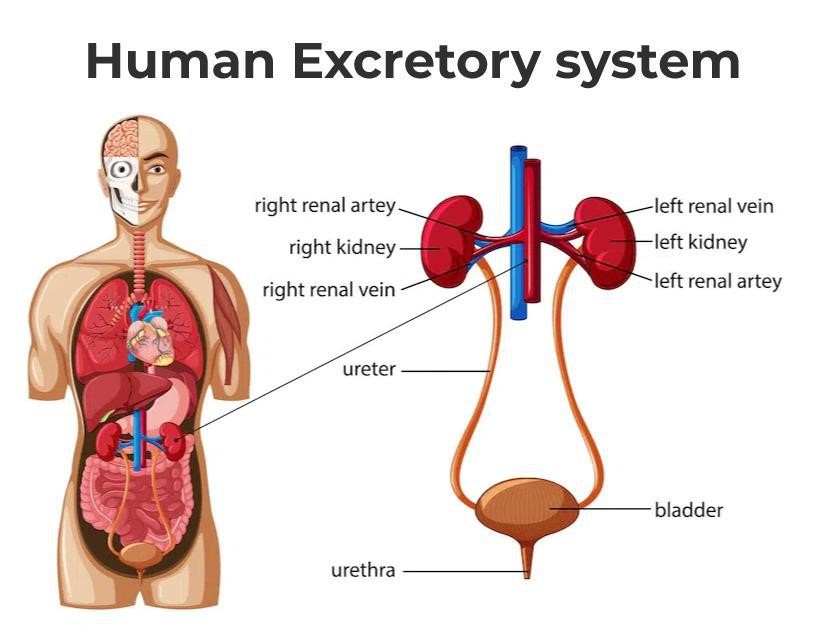
Development of the Excretory System
The development of the excretory system is a sequential process that begins early in embryogenesis and continues throughout fetal development. The excretory system develops from the intermediate mesoderm, which gives rise to three successive structures: the pronephros, mesonephros, and metanephros.
Pronephros
The pronephros is the first and most primitive form of the kidney, appearing around the fourth week of gestation. It consists of a series of paired, segmented tubules, called nephrotomes, connected by a common pronephric duct. It is a transient structure and is largely nonfunctional in humans, regressing soon after its formation. Despite its transient nature, the pronephros is important in initiating the formation of the more advanced kidney structures.
Mesonephros
The mesonephros develops after the regression of the pronephros and serves as a temporary excretory organ during early fetal development. It consists of a series of paired tubules that are more complex than those in the pronephros. These tubules are arranged in a series along the length of the mesonephric duct (also known as the Wolffian duct). Each mesonephric tubule is connected to a glomerulus, a network of capillaries that filters the blood. The filtrate is collected in the mesonephric duct and eventually transported out of the body. By the end of the first trimester, the mesonephros regresses and is replaced by the metanephros. However, the mesonephric ducts contribute to the formation of structures in the male reproductive system.
Metanephros
The metanephros is the definitive kidney, beginning to form around the fifth week of gestation and becoming fully functional during the fetal period. The metanephros develops from two sources: the metanephric mesenchyme and the ureteric bud. The ureteric bud undergoes extensive branching to form the collecting ducts and renal pelvis, while the metanephric mesenchyme differentiates into nephrons, the functional units of the kidney. As the metanephros matures, it undergoes extensive branching morphogenesis, leading to the formation of the renal pelvis, calyces, and the intricate network of nephrons that filter blood and produce urine.
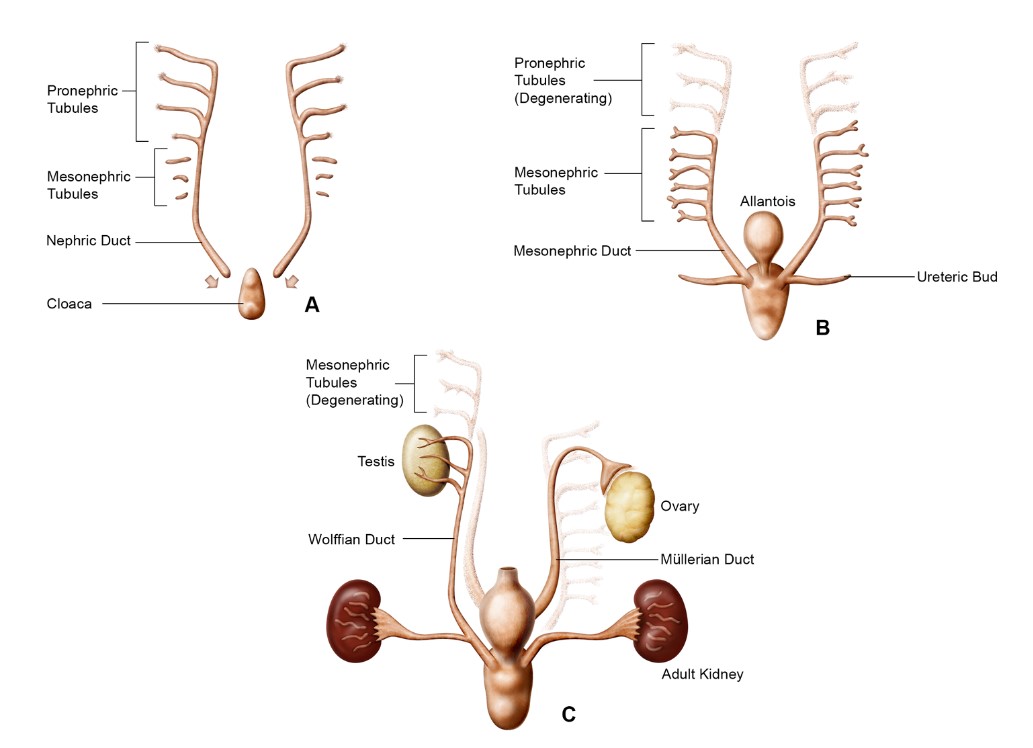
Development of the Ureters, Bladder, and Urethra
During the early stages of urinary tract development, around the fourth week of gestation in humans and embryos, an epithelial outgrowth known as the ureteric bud (UB) emerges from the Wolffian duct. This bud extends into the adjacent mesenchymal tissue called the metanephric mesenchyme. As development continues, the ureter elongates and the kidney, or metanephros, begins to form. The common nephric ducts undergo a process of shortening, dilation, and eventual integration into the urogenital sinus, which will become the future bladder.
In the mature urinary tract, urine flows from the renal pelvis of the kidney through the ureter to the bladder for storage and then is expelled through the urethra. The ureter connects to the kidney at the ureteropelvic junction (UPJ) and to the bladder at the ureterovesical junction (UVJ). Inside the bladder, the ureters open through two ureteric orifices, and the internal urethral orifice contributes to the formation of the trigone. The urethral sphincter complex consists of the lissosphincter, a continuation of the smooth muscle of the bladder, and the rhabdosphincter, which is composed of striated muscle. A transverse section of the mature ureter shows its layered structure, including the urothelium, stromal cells, smooth muscle cells, and adventitial fibroblasts.
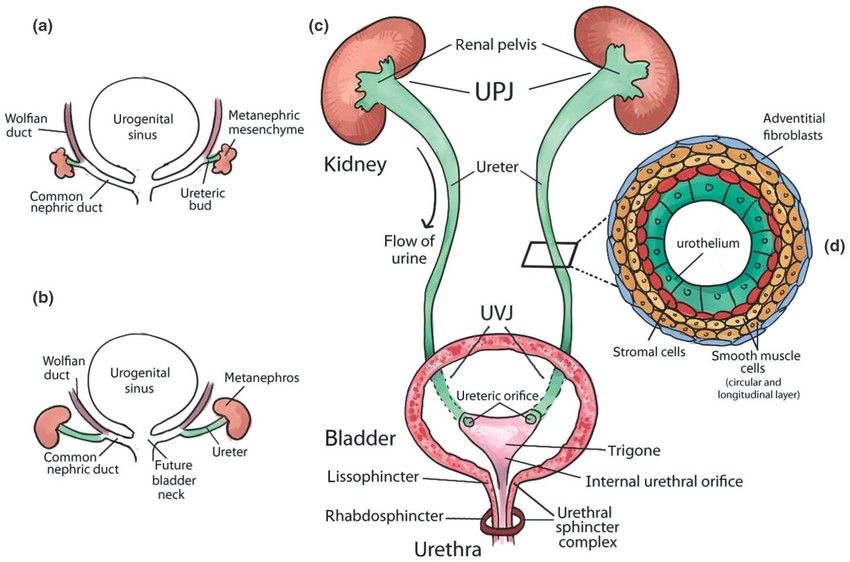
Regulation of Excretory System Development
The development of the excretory system is tightly regulated by a network of genetic and molecular signals that ensure the proper formation, differentiation, and maturation of the kidneys, ureters, bladder, and urethra. Key regulatory mechanisms include:
Inductive Interactions Between Tissues
The development of the kidneys, particularly the metanephric system, is driven by reciprocal inductive interactions between the metanephric mesenchyme and the ureteric bud. Signals from the metanephric mesenchyme induce the ureteric bud to branch and form the collecting system, while signals from the ureteric bud induce the metanephric mesenchyme to differentiate into nephrons.
Growth Factors and Signaling Pathways
GDNF/RET Signaling: Glial cell line-derived neurotrophic factor (GDNF) produced by the metanephric mesenchyme binds to the RET receptor on the ureteric bud, promoting its growth and branching. This signaling pathway is crucial for the initiation of kidney development and the formation of the ureters.
Wnt Signaling: The Wnt signaling pathway, particularly Wnt4 and Wnt11, is involved in nephron formation and the branching morphogenesis of the ureteric bud. Wnt4 is required for the mesenchymal-to-epithelial transition that forms the nephrons.
BMP (Bone Morphogenetic Protein) Signaling: BMP4 and BMP7 are important for the regulation of ureteric bud branching and the maintenance of the metanephric mesenchyme. BMP signaling also plays a role in the differentiation of the urogenital sinus into the bladder and urethra.
Transcriptional Regulation
The expression of specific transcription factors is critical for the regulation of genes involved in the development of the excretory system. These transcription factors control the differentiation of progenitor cells into the various cell types that make up the kidneys, ureters, and bladder.
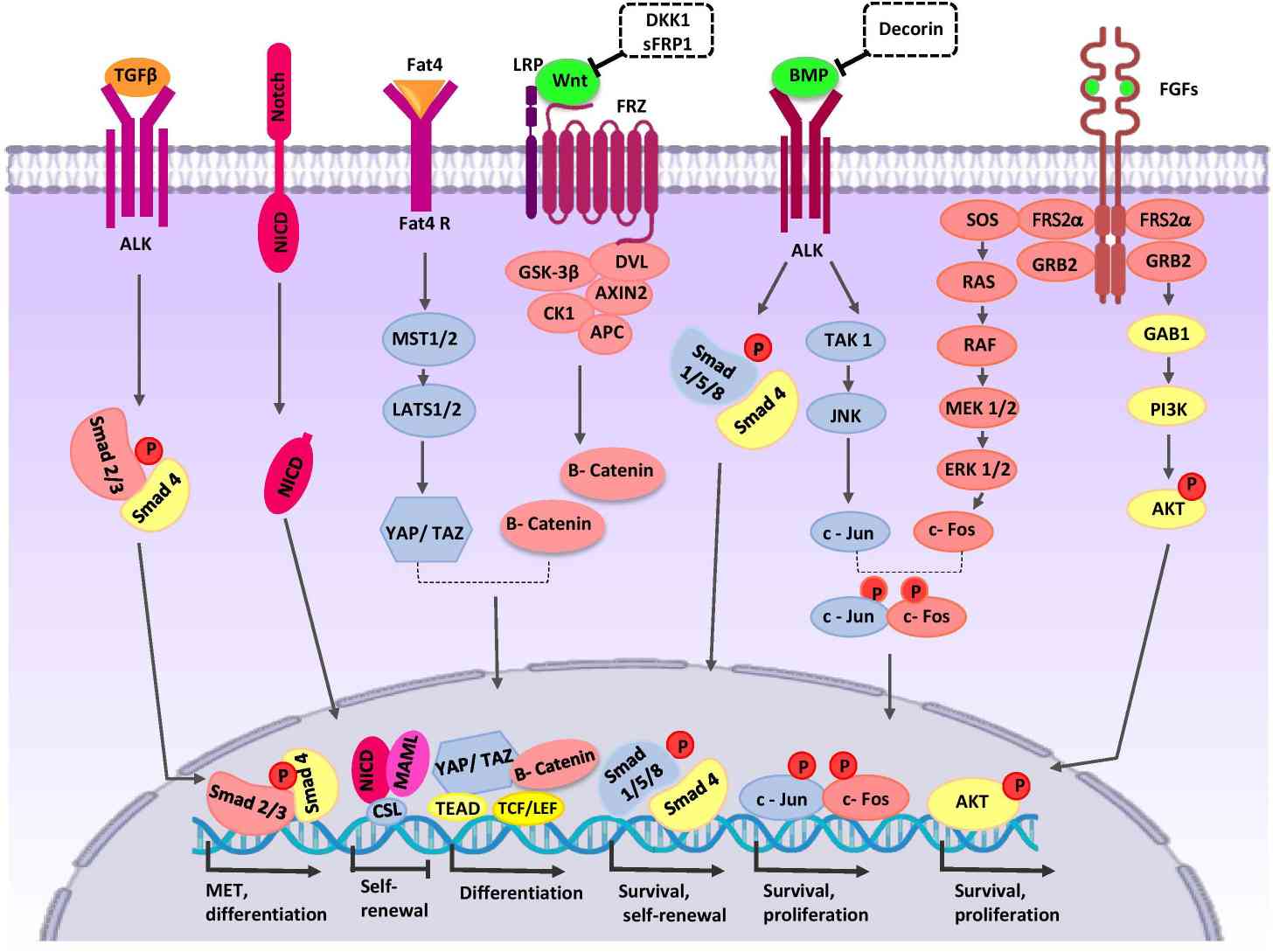
Important Transcription Factors and Cell Markers
Several transcription factors and cell markers play crucial roles in the development of the excretory system:
PAX2 and PAX8
PAX2 and PAX8 are essential for the development of the kidneys and urinary tract. PAX2 is involved in the early specification of the intermediate mesoderm and the formation of the mesonephric and metanephric kidneys. PAX8 works alongside PAX2 to regulate the expression of genes necessary for kidney development.
WT1 (Wilms Tumor 1)
WT1 is a key transcription factor expressed in the metanephric mesenchyme. It plays a vital role in kidney development by regulating the differentiation of progenitor cells into nephrons and ensuring the survival of the metanephric mesenchyme.
GDNF/RET
GDNF (Glial cell line-derived neurotrophic factor) and its receptor RET are crucial for ureteric bud formation and branching. RET is a marker of the ureteric bud and is essential for the development of the urinary collecting system.
EYA1 (Eyes Absent 1) and SIX1
EYA1 and SIX1 are transcription factors involved in kidney and ear development. They are required for the early stages of kidney development, including the formation of the metanephric mesenchyme and the ureteric bud.
FOXD1
FOXD1 is a transcription factor expressed in the stromal cells surrounding the developing kidney. It is involved in the regulation of ureteric bud branching and renal capsule formation.
CD24 and CD133
CD24 and CD133 are cell surface markers expressed in renal progenitor cells. They are used to identify and isolate renal stem cells during development and in regenerative medicine studies.
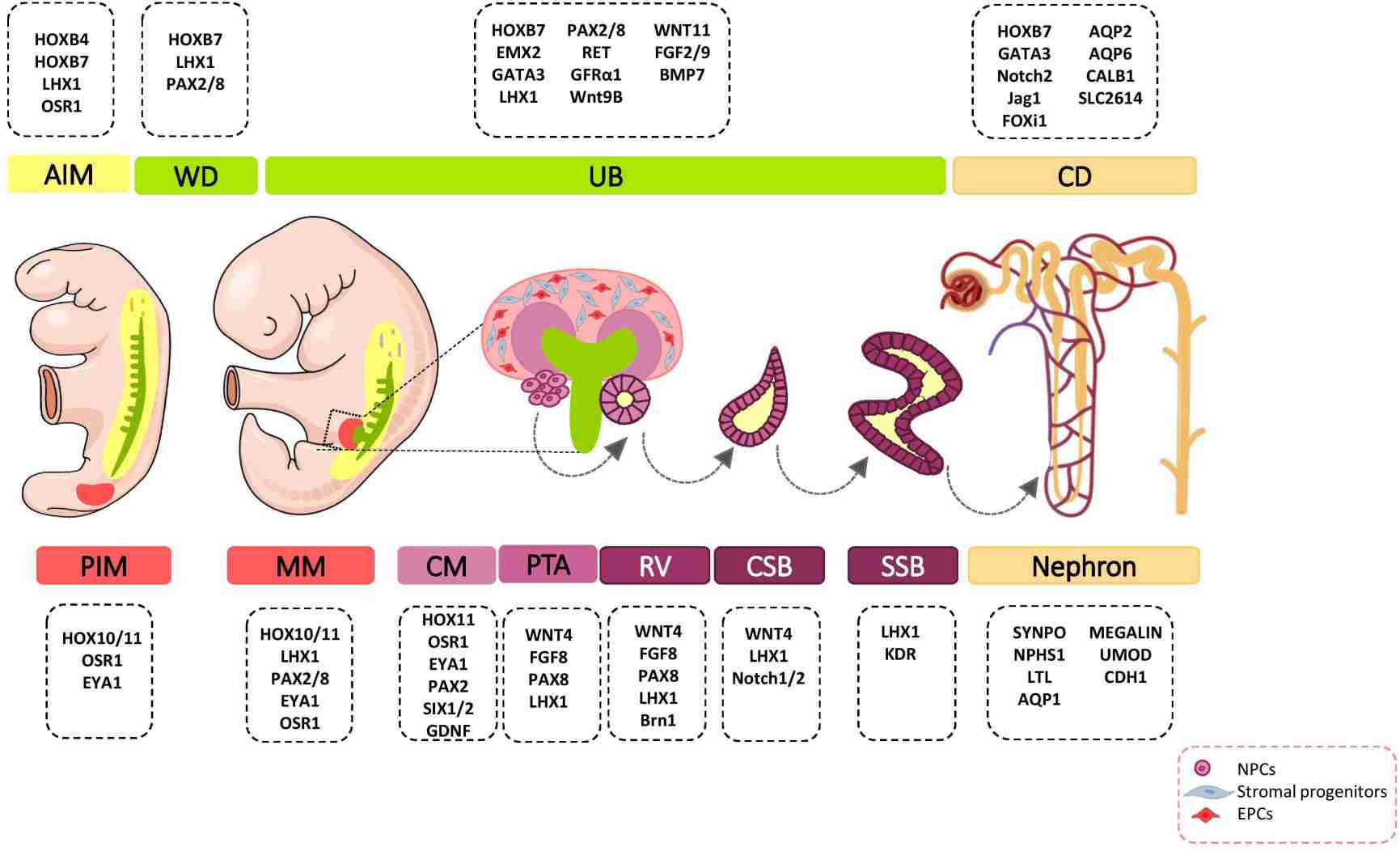
Case Study
Case 1: Michos, O.; et al. Kidney development in the absence of gdnf and spry1 requires fgf10. PLOS Genetics, 2010; 6(1), e1000809.
The study investigates the role of GDNF/RET signaling in kidney development, particularly its necessity in ureteric bud (UB) branching morphogenesis. Typically, GDNF/RET signaling is considered essential for renal organogenesis, and mutations in these genes lead to renal agenesis. However, the research shows that kidney development can still occur in the absence of Gdnf and Ret if the negative regulator Spry1 is also absent. In Gdnf-/- Spry1f-/- or Retf-/- Spry1f-/- double mutants, kidneys develop normally but with altered UB branching patterns. This development is dependent on FGF10, which takes over the role of GDNF when Spry1 is absent. However, the transcription factors ETV4 and ETV5 are irreplaceable in this signaling pathway, and their absence leads to renal agenesis that cannot be rescued by removing Spry1. The study concludes that while other signals like FGF10 can compensate for GDNF in kidney development, GDNF/RET signaling is crucial for normal UB branching patterns, and ETV4/ETV5 play an indispensable role in the RTK signaling network.
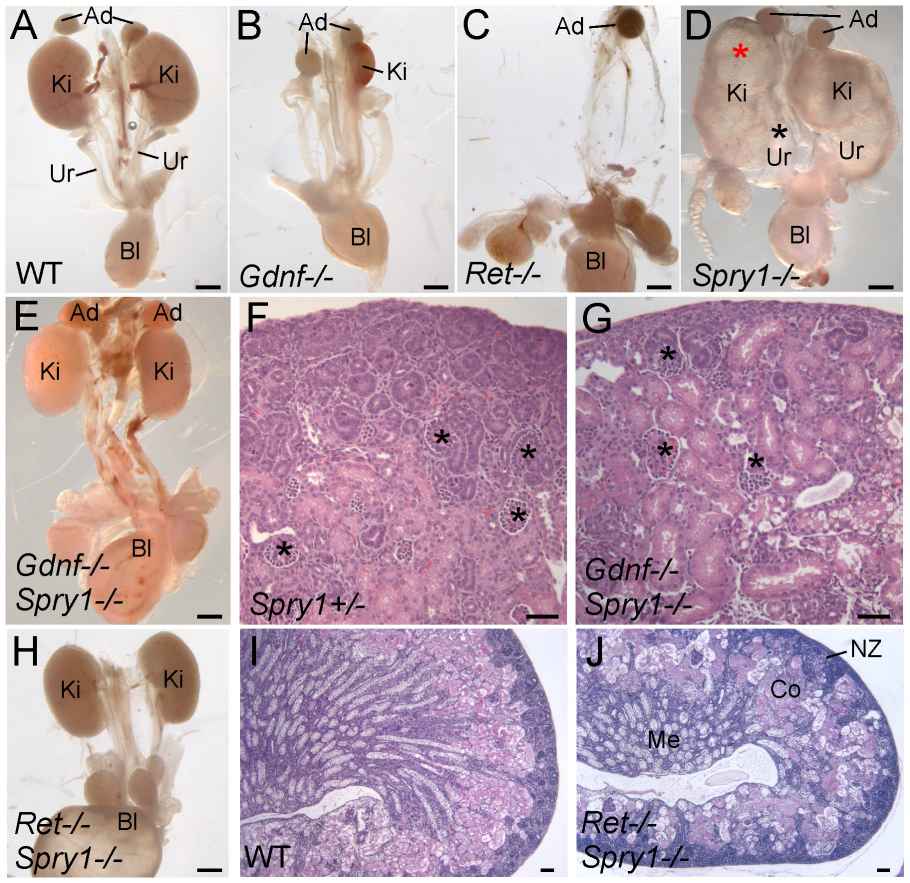
Case 2: Smeets, B.; et al. Renal progenitor cells contribute to hyperplastic lesions of podocytopathies and crescentic glomerulonephritis. Journal of the American Society of Nephrology, 2009; 20(12), 2593–2603.
This case study explores the role of renal progenitors in glomerular injury and their contribution to hyperplastic lesions in the excretory system. Glomerular injuries often lead to excessive proliferation of glomerular epithelial cells, resulting in crescent formation and the obliteration of Bowman's space. The study investigates whether the dysregulated proliferation of renal progenitors localized in Bowman's capsule contributes to these lesions. Using advanced techniques like confocal microscopy and laser capture microdissection, the study identifies three distinct cell populations in hypercellular lesions: renal progenitors (CD133+CD24+PDX-nestin-), transitional cells (CD133+CD24+PDX+nestin+), and differentiated podocytes (CD133-CD24-PDX+nestin+). The findings suggest that these lesions arise from the proliferation of renal progenitors at different stages of differentiation towards mature podocytes.
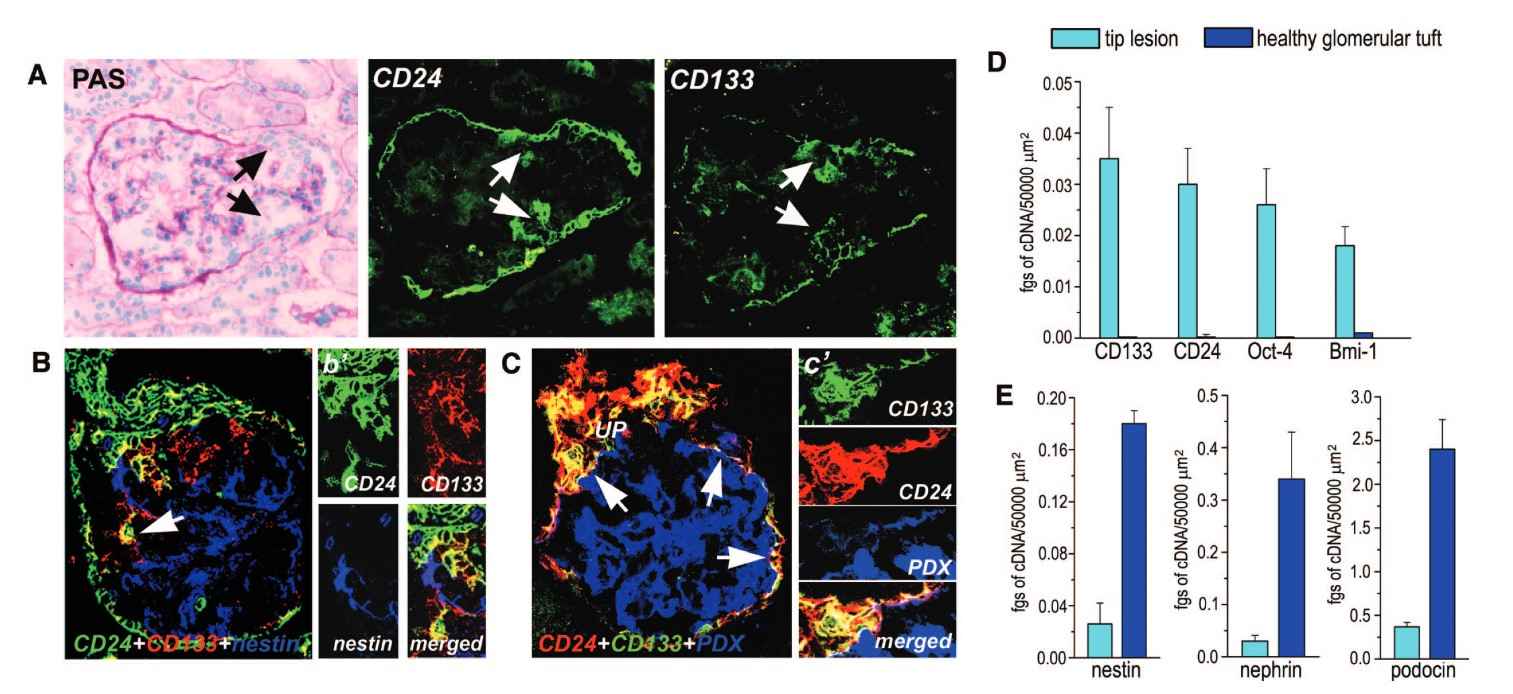
References
- Khoshdel Rad, N., Aghdami, N., & Moghadasali, R. (2020). Cellular and molecular mechanisms of kidney development: From the embryo to the kidney organoid. Frontiers in Cell and Developmental Biology, 8.
- Michos, O., Cebrian, C., Hyink, D., Grieshammer, U., Williams, L., D'Agati, V., Licht, J. D., Martin, G. R., & Costantini, F. (2010). Kidney development in the absence of gdnf and spry1 requires fgf10. PLOS Genetics, 6(1), e1000809.
- Rasouly, H. M., & Lu, W. (2013). Lower urinary tract development and disease. WIREs Systems Biology and Medicine, 5(3), 307–342.
- Seely, J. C. (2017). A brief review of kidney development, maturation, developmental abnormalities, and drug toxicity: Juvenile animal relevancy. Journal of Toxicologic Pathology, 30(2), 125–133.
- Smeets, B., Angelotti, M. L., Rizzo, P., Dijkman, H., Lazzeri, E., Mooren, F., Ballerini, L., Parente, E., Sagrinati, C., Mazzinghi, B., Ronconi, E., Becherucci, F., Benigni, A., Steenbergen, E., Lasagni, L., Remuzzi, G., Wetzels, J., & Romagnani, P. (2009). Renal progenitor cells contribute to hyperplastic lesions of podocytopathies and crescentic glomerulonephritis. Journal of the American Society of Nephrology, 20(12), 2593–2603.