Axon Guidance and Neuron Migration
Creative BioMart Axon Guidance and Neuron Migration Product List
Immunology Background
Background
Axon guidance and neuron migration are fundamental processes in the development of the nervous system. These mechanisms are critical for establishing accurate neuronal connections, which are essential for proper brain function and neural circuit formation. Axon guidance directs the growth of axons to their target regions, while neuron migration ensures that neurons reach their appropriate locations within the brain and spinal cord. Understanding these processes provides insights into neural development and can aid in addressing neurological disorders resulting from developmental abnormalities.
Structure of Neurons and Axons
Neurons are highly specialized cells responsible for transmitting information throughout the nervous system. They consist of several key structures: the cell body (soma), dendrites, and the axon. The soma contains the nucleus and organelles essential for neuronal function and metabolism. Dendrites are branched extensions that receive synaptic inputs from other neurons. The axon is a long, slender projection that carries electrical impulses from the cell body to other neurons or effector cells.
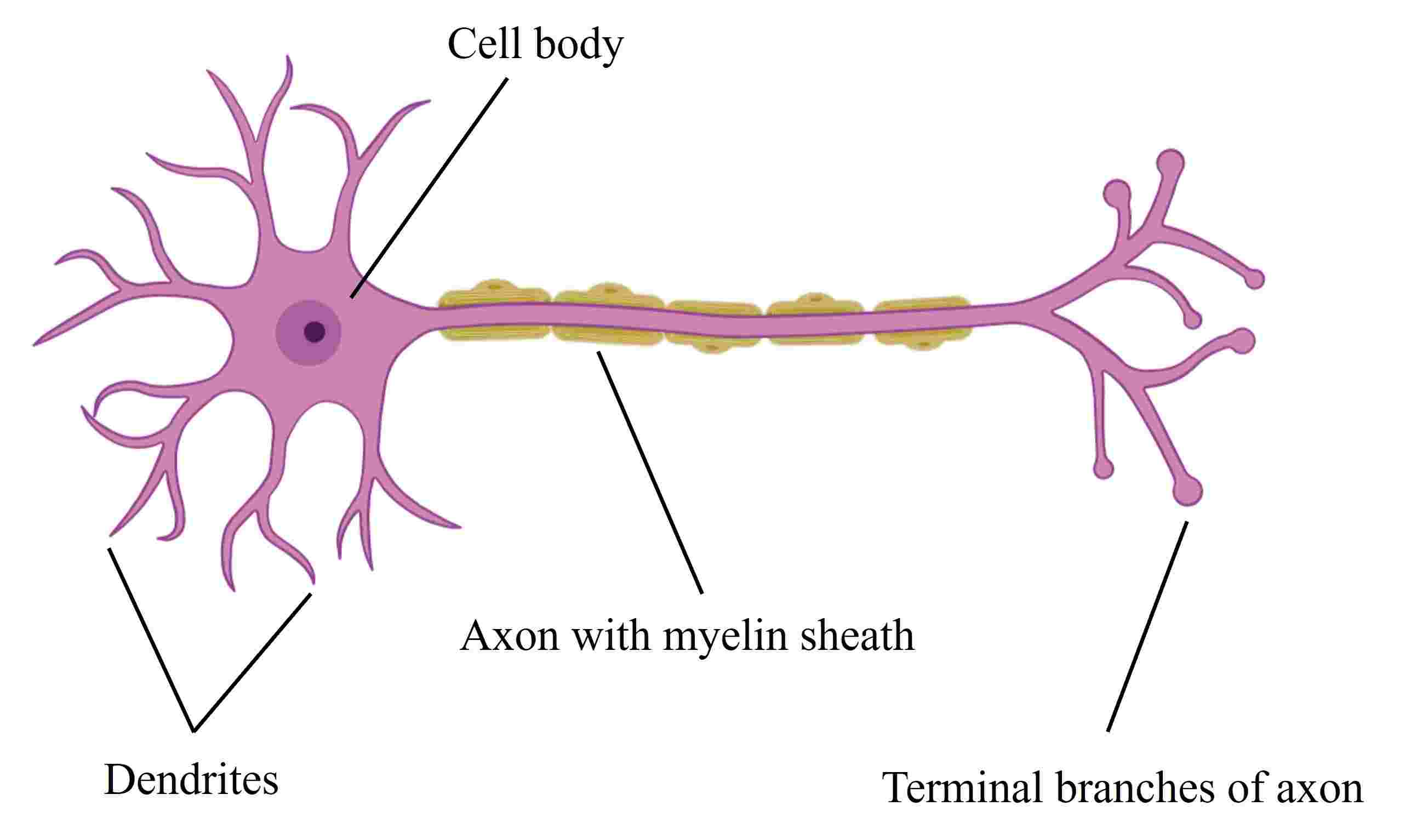
The axon is characterized by its myelin sheath, a fatty layer that insulates and accelerates electrical signal transmission. It extends from the axon hillock, a specialized region of the cell body, and terminates in axon terminals that synapse with target cells. The growth cone, a dynamic, finger-like structure at the tip of the axon, plays a critical role in exploring the environment and directing axon growth to target areas.
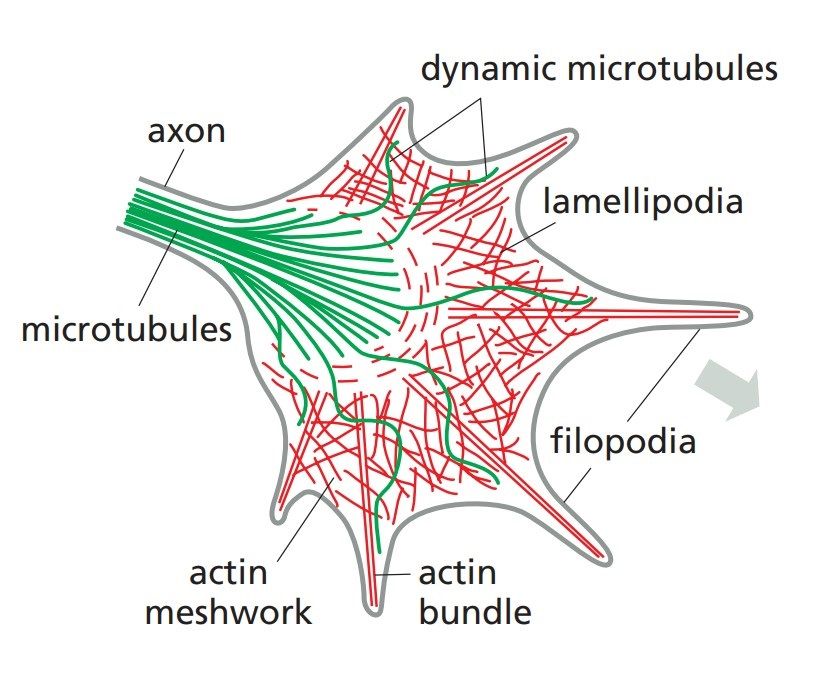
Axon Guidance
Axon guidance involves the processes by which growing axons are directed to their appropriate targets. This guidance is mediated by a complex interplay of attractive and repulsive cues in the axon environment. Chemotactic signals are soluble molecules that create gradients in the extracellular environment that attract or repel growing axons. For example, netrins attract axons through their receptors, while slit proteins repel them. Cell adhesion molecules (CAMs), such as cadherins and integrins, facilitate interactions between axons and the extracellular matrix or other cells, thereby guiding axonal growth. Ephrin-Eph receptor interactions mediate bidirectional signaling that regulates axon directionality and border formation.
Important Axon Guidance Molecules
The four major classes of axon guidance molecules are netrins, ephrins, slits, and semaphorins, each of which plays a distinct role in this process.
Netrins: Netrins are a family of secreted guidance cues that include netrin-1 and netrin-2. They attract or repel growing axons depending on the receptors to which they bind. In general, netrins attract axons through their receptors (e.g., DCC) and guide them to their targets. In some contexts, netrins can also repel axons through other receptors (e.g., UNC5).
Ephrins: Ephrins are a family of cell surface molecules that interact with Eph receptors on adjacent cells. Ephrins and Eph receptors mediate bidirectional signaling that affects the growth cone's direction. Ephrin-Eph interactions typically act as repulsive signals to prevent axons from crossing certain boundaries, thus helping to establish precise topographical maps and boundaries in the nervous system.
Slits: Slits are a family of secreted proteins that include Slit1, Slit2, and Slit3. Slits primarily act as repulsive guidance cues. They interact with Robo receptors on the growth cones of axons to prevent them from crossing midline structures or migrating inappropriately. This helps to establish correct neural pathways and ensure proper axonal guidance.
Semaphorins: Semaphorins are a diverse family of secreted and membrane-bound proteins, such as semaphorin 3A, 3F, and 4D. Semaphorins generally act as repulsive signals to guide axons away from certain areas or structures. They interact with neuropilin and plexin receptors on growth cones to regulate cytoskeletal dynamics and axon guidance, influencing both growth direction and axon branching.
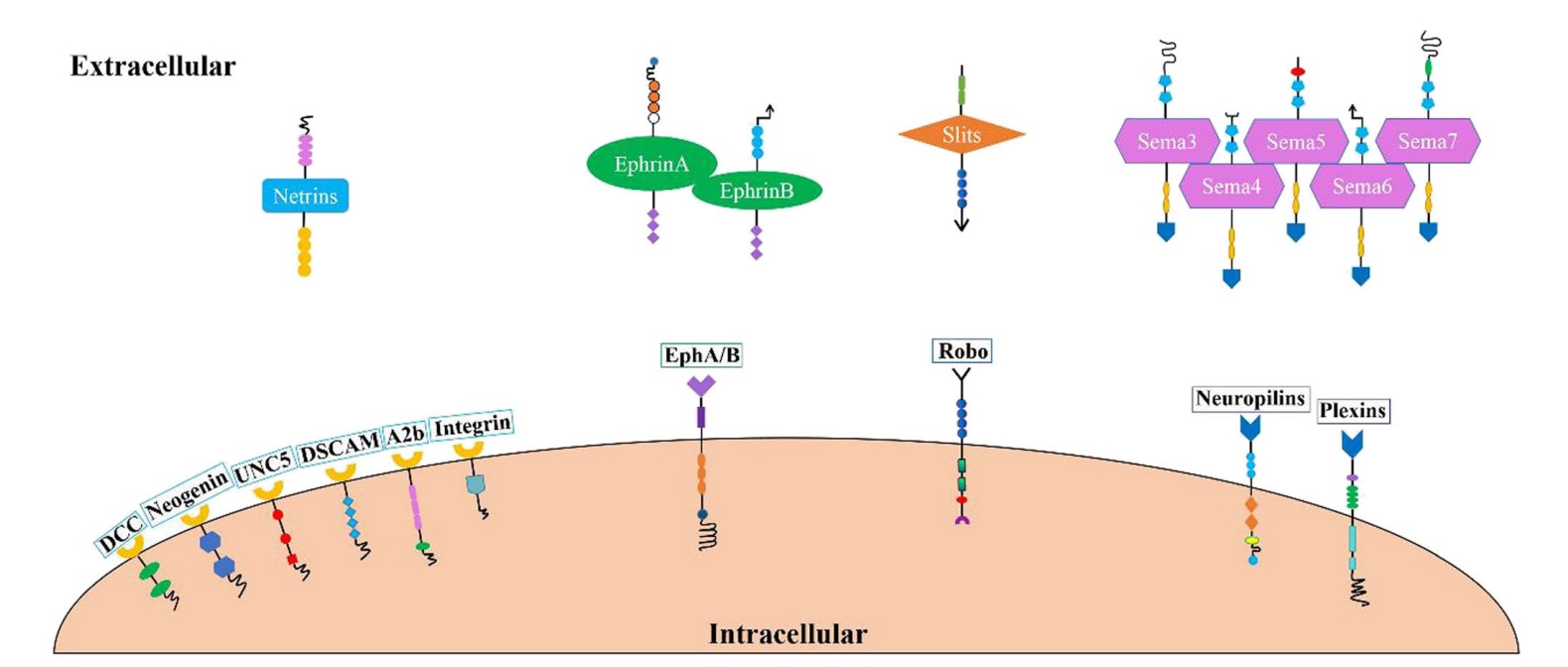
Neuron Migration
Neuron migration is a critical process in the development of the nervous system, involving the movement of neurons from their point of origin to their final destination within the brain and spinal cord. This migration is essential for the proper formation and organization of neural circuits, which underlie proper brain function and neural connectivity. Neuronal migration occurs through several key mechanisms:
Radial Migration: This mechanism is fundamental to the development of the cerebral cortex. Neurons originating in the ventricular zone migrate along radial glial fibers that serve as scaffolds. These glial fibers extend from the ventricular zone to the cortical plate, guiding neurons in a radial direction. As neurons migrate, they sequentially populate the cortical layers, with earlier-born neurons settling in deeper layers and later-born neurons occupying more superficial layers. This process ensures the proper layering and organization of the cortex, which is critical for proper cognitive function.
Tangential Migration: In contrast to radial migration, tangential migration involves the movement of neurons parallel to the ventricular surface. This type of migration is important for positioning certain types of neurons, such as interneurons, that originate from different regions and need to be integrated into the cortical network. Tangential migration allows these neurons to reach their appropriate locations in different cortical areas and other regions of the brain, contributing to the functional diversity and connectivity of neural circuits.
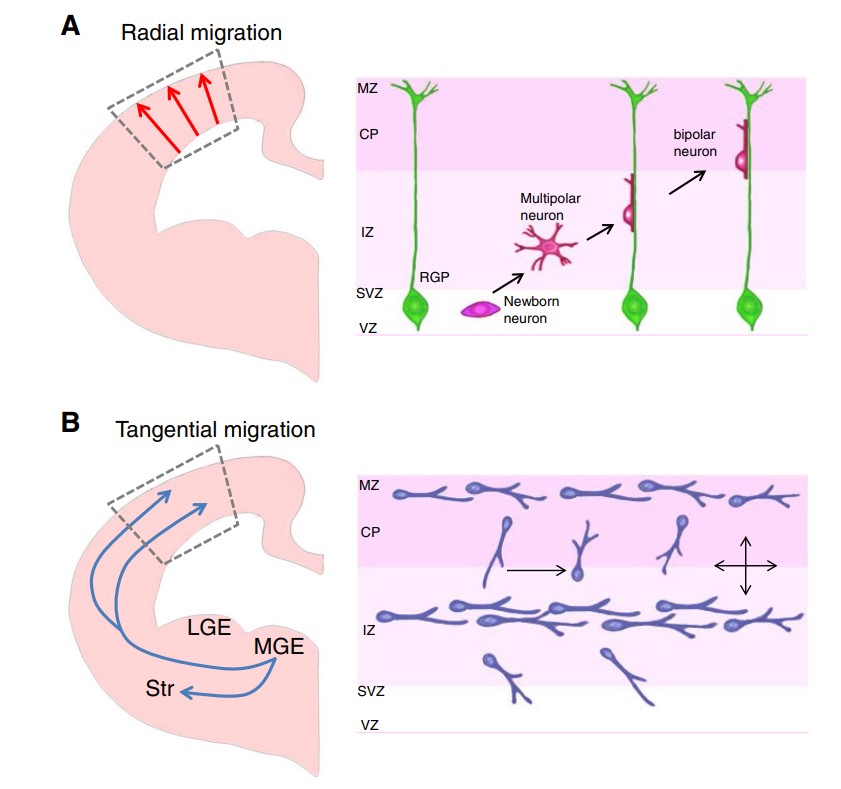
Crawling: Neuronal crawling is a process by which neurons extend their leading processes, adhere to extracellular matrix components or cellular substrates, and then retract their trailing processes to advance forward. This mechanism involves dynamic changes in the cytoskeleton, particularly actin filaments, which drive the movement of the growth cone and cell body. Neuronal crawling is critical for fine-tuning the positioning of neurons and enabling their integration into complex networks. It also plays a role in guiding neurons through confined spaces or along specific pathways within the developing brain.
Important Neuron Migration Molecules
Neuron migration involves a variety of important molecules that guide and regulate the movement of neurons during development. These molecules can be categorized into several key types:
Guidance Cues
The four major classes of axon guidance molecules also play a role in neuronal migration:
Netrins act as attractants or repellents to guide neurons to their destinations. Netrin-1 binds to Deleted in Colorectal Cancer (DCC) receptors to attract neurons and to UNC5H receptors to repel them.
Ephrins and Eph receptors provide bidirectional guidance cues, with EphA receptors and Ephrin-A ligands interacting to guide neurons. Ephrin-B ligands interact with EphB receptors to influence neuronal positioning and migration.
Slits primarily act as repellents to prevent inappropriate migration. Slit2 binds to Robo (Roundabout) receptors to regulate axon guidance and prevent axons from crossing midline structures.
Semaphorins can either repel or attract neurons depending on the receptor context. Semaphorin 3A binds to neuropilin-1 and plexin-A1 receptors to repel migrating neurons.
Adhesion Molecules
Integrins are critical for neuronal attachment to the extracellular matrix (ECM), facilitating migration during brain development. These transmembrane receptors link neurons to ECM proteins such as fibronectin. Specifically, integrin α5β1 binds to fibronectin, enabling neurons to move by providing the necessary traction and directional signals. This interaction not only anchors neurons, but also triggers intracellular pathways that regulate their movement, ensuring that they reach their destination.
Cadherins, particularly N-cadherin, are essential for cell-cell adhesion, which is critical for neuronal alignment and migration. N-cadherin mediates interactions between migrating neurons and radial glial cells, guiding neurons to their proper positions in the developing cortex. This adhesion is critical for proper cortical layer formation and neural circuit development. Disruptions in N-cadherin function can lead to misaligned neurons and potential neurological problems.
Cell Adhesion Molecules (CAMs) such as NCAM (Neural Cell Adhesion Molecule) play a key role in promoting neuronal adhesion and migration. NCAM supports neurite outgrowth and facilitates cell-cell interactions that are critical for guiding migrating neurons to their destinations. By stabilizing these connections, CAMs ensure proper neural network formation, which is essential for functional brain development.
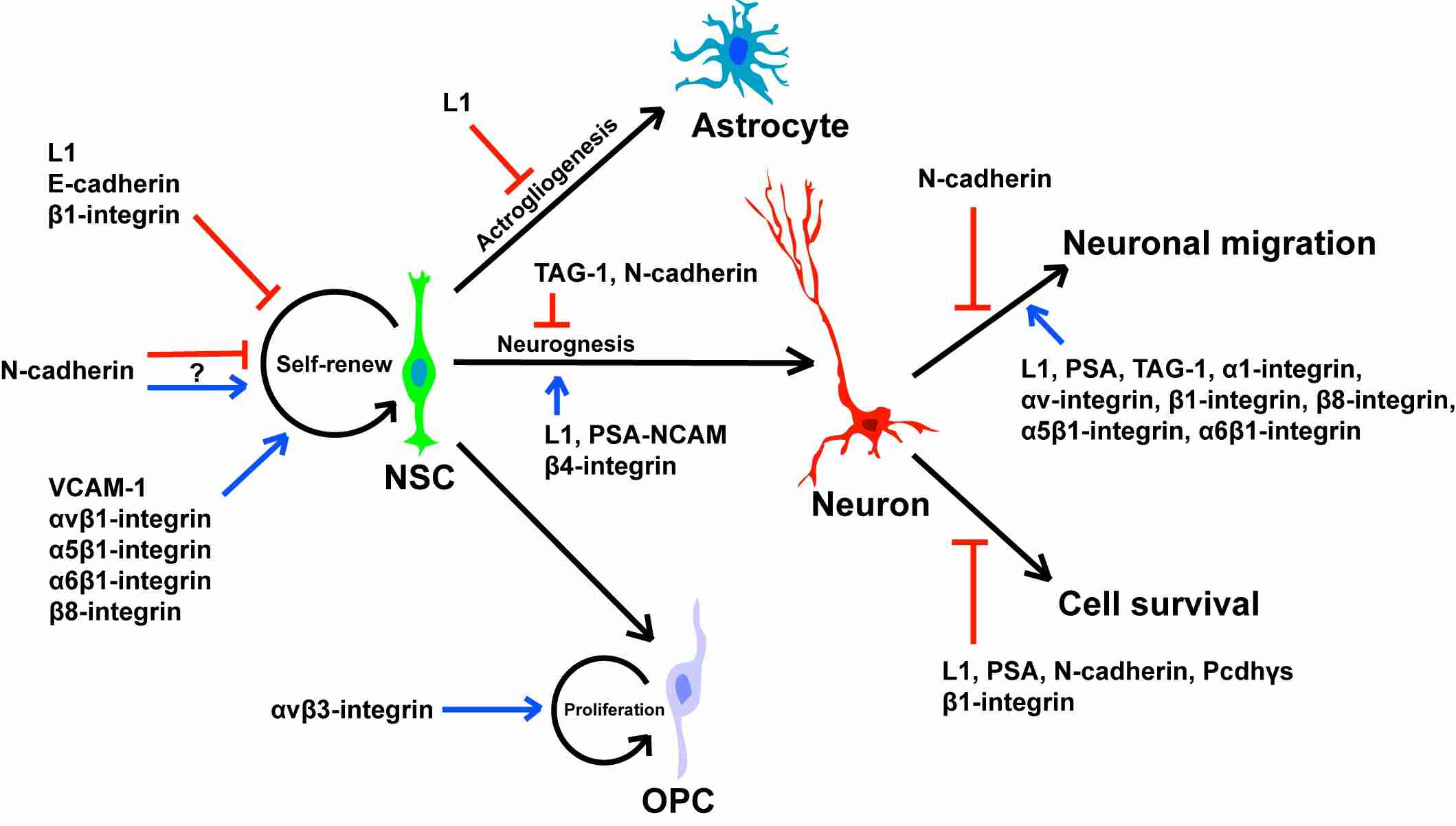
Extracellular Matrix (ECM) Proteins
Extracellular matrix (ECM) proteins such as laminin and fibronectin play a role in neuronal migration:
Laminin is a critical component of the extracellular matrix (ECM) that plays an important role in neuronal adhesion and migration. It provides a supportive substrate for neurons to adhere to as they migrate to their destinations during brain development. Laminin-1, a specific isoform of laminin, is particularly important in this process. It interacts with integrins on the surface of neurons and serves as a guide for their movement. This binding triggers intracellular signaling pathways that affect the cytoskeleton, allowing neurons to extend their processes and migrate effectively. In addition to supporting neuronal adhesion, laminin also helps guide neurons along specific pathways, ensuring that they reach the correct regions within the brain and contribute to the formation of functional neural circuits.
Fibronectin is another crucial ECM protein that supports neuronal movement by interacting with integrins on the surface of migrating neurons. This interaction plays a key role in facilitating neuronal migration across the ECM, providing both structural support and directional cues. By binding to integrins such as integrin α5β1, fibronectin helps anchor neurons to the ECM, allowing them to generate the necessary traction to move forward. This process is essential for the proper positioning of neurons during development, contributing to the organization of neural tissues and the establishment of functional connections in the nervous system. The role of fibronectin in neuronal migration underscores its importance in brain development and the formation of complex neural networks.
Cytoskeletal Regulators
Rho GTPases are a family of small signaling proteins that play a critical role in regulating cytoskeletal dynamics, thereby influencing neuronal movement. These molecules act as molecular switches that control the organization and behavior of actin filaments, which are essential for cell shape and motility. Among the Rho GTPases, Rac1 and Cdc42 are particularly important for neuronal migration. Rac1 is involved in the formation of lamellipodia, sheet-like projections at the leading edge of migrating neurons. By promoting the polymerization of actin filaments, Rac1 drives the forward movement of these protrusions, helping neurons to advance toward their targets. Similarly, Cdc42 regulates the formation of filopodia, slender, finger-like extensions that probe the environment. Together, Rac1 and Cdc42 coordinate the actin cytoskeleton, enabling neurons to navigate through the extracellular matrix and reach their proper destinations in the developing nervous system.
Myosin and actin filaments are key players in driving neuronal movement through their interactions within the cytoskeleton. Actin filaments, which are dynamic protein structures, provide the structural framework that supports the extension of neuronal processes such as lamellipodia and filopodia. Myosin II, a motor protein, works in concert with actin filaments to generate the force necessary for movement. It does this by binding to actin and using energy from ATP hydrolysis to generate mechanical work, resulting in contraction of the actin network. This contraction allows neurons to pull themselves forward by retracting their trailing edge while extending their leading edge. The coordinated action of myosin II and actin filaments is essential for the precise and directed migration of neurons during brain development, ensuring that they reach their designated locations and contribute to the formation of functional neural circuits.
Signaling Pathways
The Wnt signaling pathway is critical for neuronal proliferation, differentiation and migration, primarily mediated by β-catenin. Wnt3a, a key ligand, significantly influences the migration of neural progenitor cells in the developing cortex. When Wnt3a activates its receptor, it stabilizes β-catenin, which then translocates to the nucleus to regulate genes involved in cell movement. This process ensures that neural precursors reach their correct locations, contributing to the layered structure of the brain and the proper formation of neural circuits.
The Notch signaling pathway is essential for the balance of neuronal and glial cell development, influencing both differentiation and migration. Activation of Notch leads to the release of its intracellular domain (NICD), which enters the nucleus and regulates gene expression. This process determines whether a progenitor cell will become a neuron or a glial cell and influences cell positioning by controlling genes involved in cytoskeletal dynamics and adhesion. Notch ensures that cells are properly positioned and contributes to functional neural networks.
Growth Factors and Cytokines
Brain-Derived Neurotrophic Factor (BDNF) plays an important role in the nervous system by supporting neuronal survival, growth and migration. BDNF exerts its effects primarily by binding to Tropomyosin receptor kinase B (TrkB) receptors on the surface of neurons. Upon binding, BDNF activates intracellular signaling pathways that promote neuronal survival under stress conditions, promote dendrite and axon growth, and enhance synaptic plasticity, which is essential for learning and memory. In addition, BDNF facilitates the migration of neurons during development, ensuring that they reach their proper locations in the brain. This trophic support is critical for the development and maintenance of a healthy nervous system and is involved in several processes, including neural circuit formation and response to injury.
Transforming Growth Factor-beta (TGF-β) is a key regulator of neuronal migration and differentiation and has a major impact on the development of the nervous system. TGF-β influences these processes by modulating gene expression through its signaling pathway. When TGF-β binds to its receptors, it activates SMAD proteins, which translocate to the nucleus to regulate the transcription of target genes. This regulation is particularly important in directing the migration of oligodendrocyte precursor cells, which are critical for the formation of myelin in the central nervous system. TGF-β signaling ensures that these precursor cells migrate to the correct locations and differentiate into mature oligodendrocytes, thereby contributing to proper insulation of neuronal axons and efficient signaling.
Case Study
Case 1: Lisabeth, E. M.; et al. Eph receptor signaling and ephrins. Cold Spring Harbor Perspectives in Biology, 2013; 5(9), a009159–a009159.
Eph receptors, members of the receptor tyrosine kinase (RTK) family, play a critical role in cell communication by interacting with ephrin ligands on neighboring cells. Unlike other RTKs that bind to soluble ligands, Eph receptors facilitate contact-dependent signaling that results in bidirectional communication between cells. This system is essential for various developmental processes and the maintenance of adult tissue homeostasis. Eph receptors and ephrins are widely expressed, particularly in the brain, where they regulate processes such as axon guidance, synapse formation, and tissue organization. Dysregulation of the Eph/ephrin system has been implicated in several diseases, including cancer, making these receptors potential therapeutic targets.
Eph receptor signaling involves the formation of receptor clusters upon binding to ephrin ligands. These clusters can include both ephrin-bound and unbound Eph receptors, leading to trans-phosphorylation and activation of the kinase domain. Clustering is influenced by the cellular context, such as association with the actin cytoskeleton, and involves interactions between different classes of Eph receptors. This process results in the recruitment of several downstream signaling proteins, including Rho and Ras family GTPases and Akt/mTORC1, which are critical for signal transduction. While Eph receptors typically inhibit cell growth and promote cell repulsion, their signaling can be modulated by kinase-inactive receptors and alternative splicing, leading to diverse biological outcomes, including tumor suppression or cell adhesion.
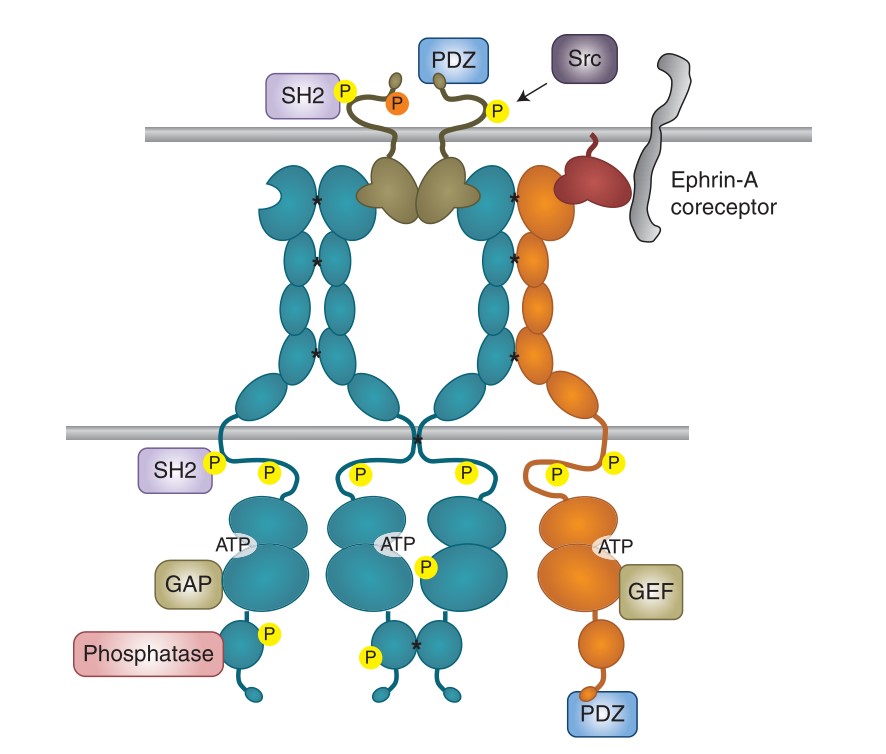
Case 2: Li, L.; et al. BDNF and NGF signals originating from sensory ganglia promote cranial motor axon growth. Experimental Brain Research, 2020; 238(1), 111–119.
This case study examines the role of sensory ganglia in promoting motor axon growth from the hindbrain. First, the study examines whether this effect is specific to certain ganglia and identifies the underlying signaling mechanisms. Using co-cultures of ventral rhombomere 8 explants with various sensory ganglia in a collagen gel matrix, the research shows that all cranial sensory ganglia, including trunk dorsal root ganglia, can enhance motor axon growth. The study also finds that older ganglia have a stronger effect on axonal growth compared to younger ganglia. Importantly, the effect is mediated by brain-derived neurotrophic factor (BDNF) and nerve growth factor (NGF), which are both necessary and sufficient for this enhancement. These results demonstrate that the axon growth-promoting effect is stage-dependent, but not specific to a particular ganglion.
The researchers functionally blocked BDNF and NGF by adding specific antibodies to the medium during co-culture of r8 ventral neural tube explants with sensory ganglia. The axon growth-promoting effect of sensory ganglia was significantly reduced when BDNF or NGF antibodies were added to the co-culture medium. Thus, neutralization of BDNF and NGF blocks the promoting function of sensory ganglia on axon growth from r8 ventral neural tube explants.
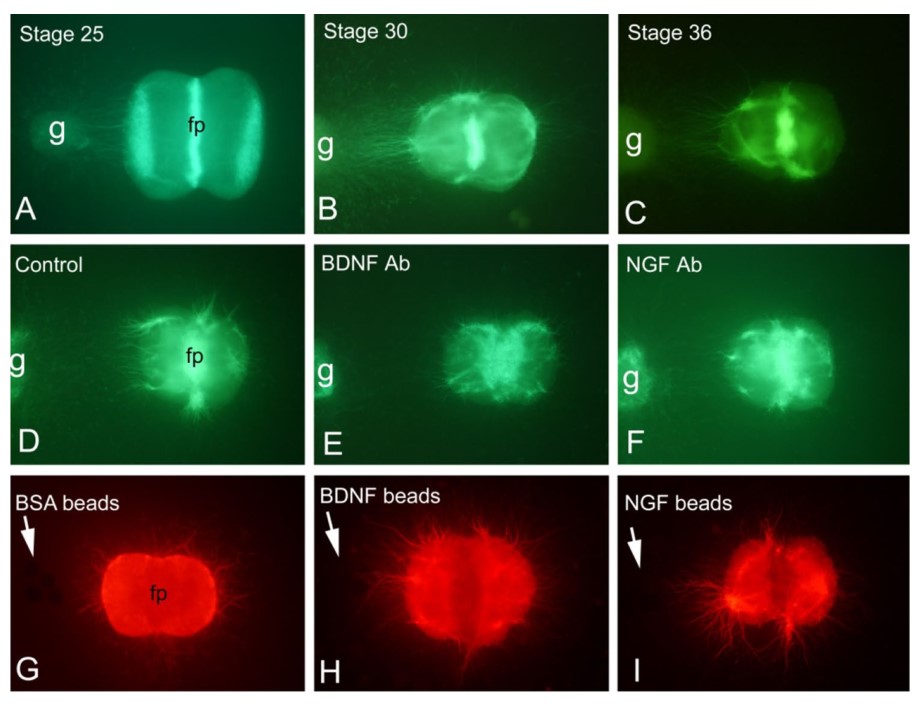
References
- Molecular biology of the cell (6th edition, 2015). Garland Science, Taylor and Francis group.
- Bian, S. (2013). Cell adhesion molecules in neural stem cell and stem cell- based therapy for neural disorders. In L. Bonfanti (Ed.), Neural Stem Cells—New Perspectives. InTech.
- Li, L., Pu, Q., Hintze, M., Wang, Y., Eckhardt, M., Gieselmann, V., Tiemann, I., Qi, X., Cai, D., Wang, J., & Huang, R. (2020). BDNF and NGF signals originating from sensory ganglia promote cranial motor axon growth. Experimental Brain Research, 238(1), 111–119.
- Lisabeth, E. M., Falivelli, G., & Pasquale, E. B. (2013). Eph receptor signaling and ephrins. Cold Spring Harbor Perspectives in Biology, 5(9), a009159–a009159.
- Moffat, J. J., Ka, M., Jung, E.-M., & Kim, W.-Y. (2015). Genes and brain malformations associated with abnormal neuron positioning. Molecular Brain, 8(1), 72.
- Zhang, L., Qi, Z., Li, J., Li, M., Du, X., Wang, S., Zhou, G., Xu, B., Liu, W., Xi, S., Xu, Z., & Deng, Y. (2021). Roles and mechanisms of axon-guidance molecules in alzheimer's disease. Molecular Neurobiology, 58(7), 3290–3307.