Muscular System Development
Creative BioMart Muscular System Development Product List
Immunology Background
Background
The muscle system is a fundamental component of the vertebrate body, responsible for producing movement, maintaining posture, and enabling various physiological functions such as breathing and circulation. Muscles are derived from mesodermal tissues during embryonic development and are classified into three main types: skeletal, smooth, and cardiac. The development of the muscle system, known as myogenesis, involves a complex interplay of signaling pathways, transcription factors, and cellular interactions that regulate the formation, growth, and maturation of muscle fibers.
Overview of the Muscular System
Muscles are classified based on their structure and function:
- Skeletal muscles are voluntary, striated muscles that attach to bones and are responsible for movement.
- Smooth muscles are involuntary, non-striated muscles found in the walls of internal organs such as the intestines and blood vessels.
- Cardiac muscles are involuntary, striated muscles that make up the heart and are specialized for continuous contraction to pump blood.
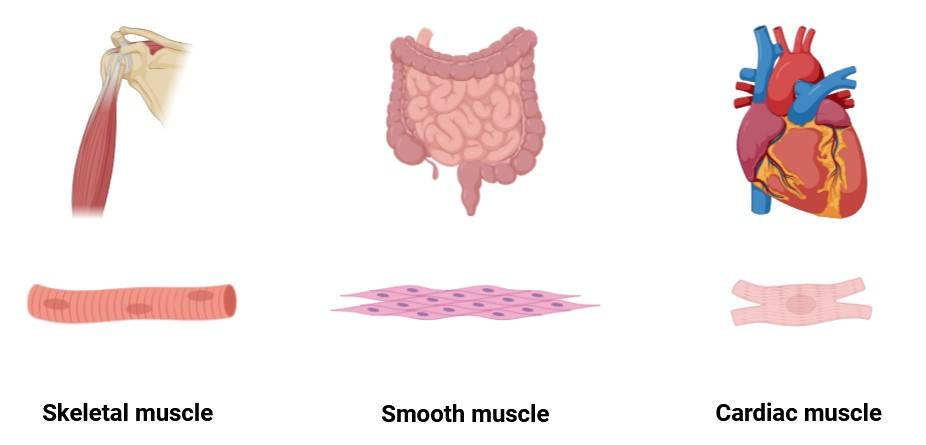
All three types of muscles arise from the mesoderm, one of the three germ layers formed during gastrulation. While each type of muscle follows a distinct developmental pathway, they share common regulatory mechanisms during early embryogenesis.
Mesodermal Origins of Muscle Tissue
The mesoderm, which forms during the early stages of embryonic development, gives rise to various tissues, including muscle, bone, and connective tissue (early mesodermal lineage markers). Specifically, the paraxial mesoderm, located on either side of the neural tube, is the source of skeletal muscle, while the lateral plate mesoderm contributes to smooth and cardiac muscle development.
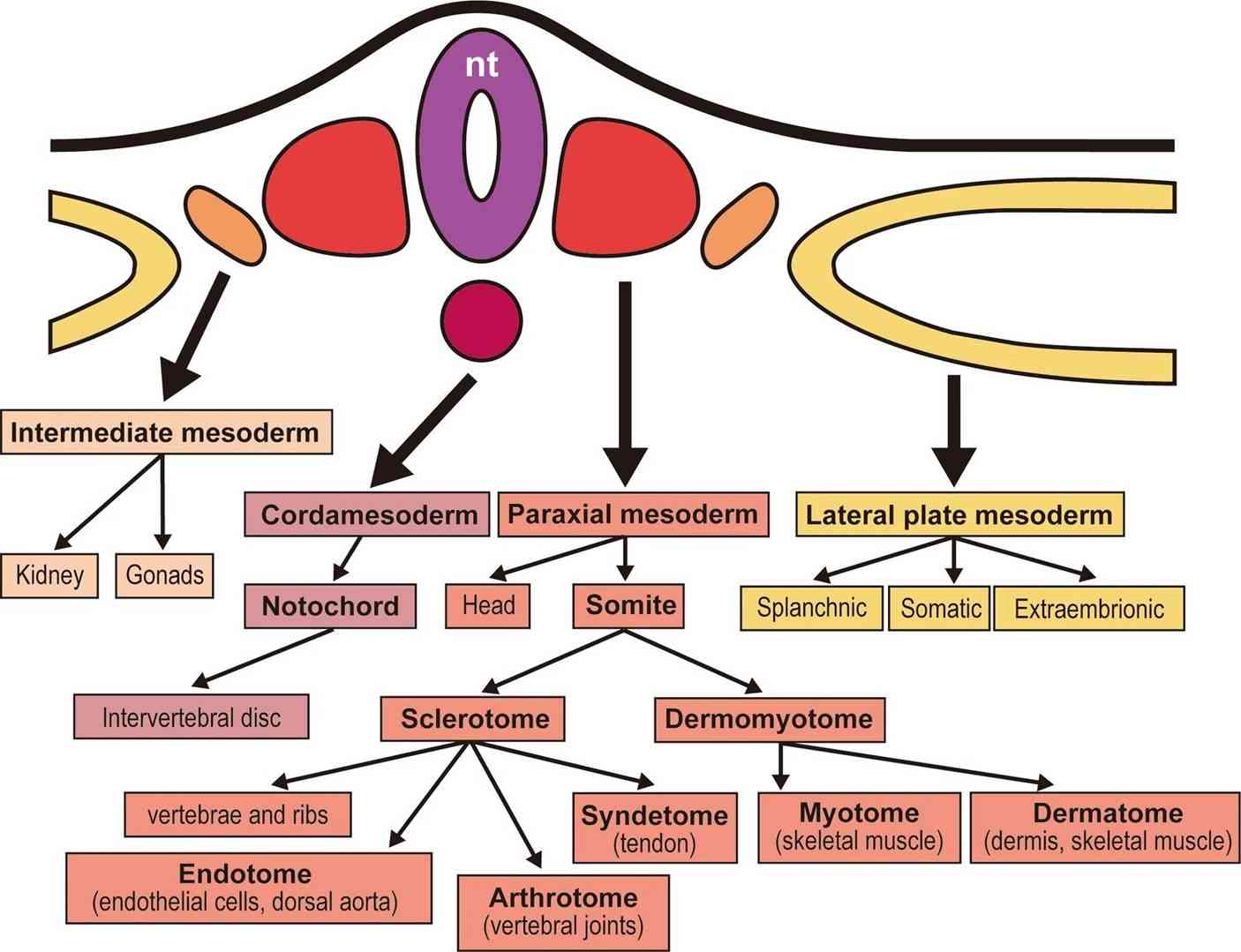
During segmentation, the paraxial mesoderm forms block of tissue called somites, which are critical for skeletal muscle formation. The somites further differentiate into two major compartments:
- The dermomyotome, which gives rise to dermis and muscle,
- The sclerotome, which forms the vertebrae and ribs.
Cells within the dermomyotome undergo further specification to form the myotome, which contains the precursors to skeletal muscle cells, known as myoblasts.
Molecular Regulation of Myogenesis
Muscle development is regulated by a cascade of transcription factors that control the differentiation of myoblasts into mature muscle fibers. The myogenic regulatory factors (MRFs) - MyoD, Myf5, myogenin and MRF4 - play a central role in this process. These factors are activated in a sequential manner and promote the progression of myogenesis from the proliferation of muscle precursors to the formation of functional muscle fibers.
- Myf5 and MyoD are expressed early in myogenesis and are essential for the commitment of progenitor cells to the muscle lineage. Myf5 is required for the specification of muscle progenitors in the somite, while MyoD further promotes their differentiation into myoblasts.
- Myogenin and MRF4 are involved in the later stages of muscle differentiation, including the fusion of myoblasts into multinucleated muscle fibers called myotubes. Myogenin is particularly important for the maturation of myotubes into functional muscle fibers.
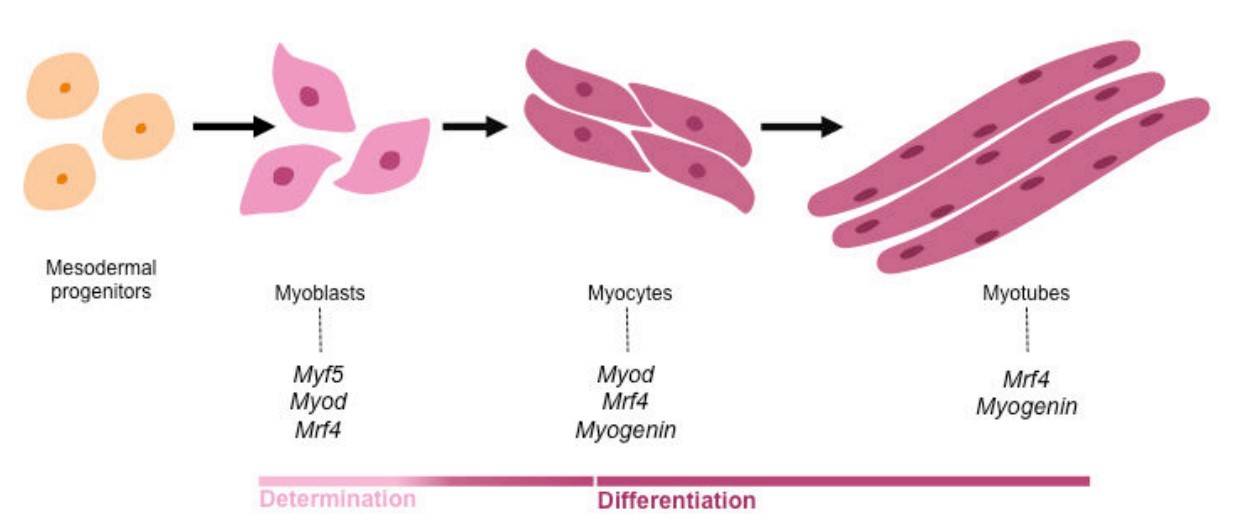
In addition to the MRFs, several other signaling pathways regulate myogenesis, including the Wnt, Notch, and Sonic Hedgehog (Shh) pathways. Wnt signaling promotes the activation of Myf5 and MyoD, while Notch signaling maintains the balance between progenitor proliferation and differentiation. Sonic Hedgehog is involved in the induction of muscle progenitors in the somite.
Skeletal Muscle Development
Skeletal muscle development occurs in several stages, beginning with the formation of somites from the paraxial mesoderm. Once the somites are formed, they undergo further differentiation into the dermomyotome and sclerotome, as mentioned above. The myogenic precursors within the dermomyotome express Pax3 and Pax7, transcription factors that regulate the maintenance of muscle precursors and their subsequent differentiation into myoblasts.
Myoblasts then proliferate and begin to express MyoD and Myf5, committing them to the muscle lineage. Following commitment, myoblasts exit the cell cycle and begin to differentiate, expressing genes required for muscle contraction, such as myosin heavy chain and actin. These myoblasts fuse to form myotubes, which further differentiate into mature, multinucleated muscle fibers.
As the embryo grows, the number of muscle fibers increases through a process called hyperplasia. Postnatally, muscle fiber growth occurs primarily through hypertrophy, or the enlargement of existing fibers. This growth is regulated by factors such as insulin-like growth factor 1 (IGF-1) and the myostatin signaling pathway. Myostatin, a member of the TGF-β family, negatively regulates muscle growth by inhibiting myoblast proliferation and differentiation.
Cardiac Muscle Development
Cardiac muscle, which forms the heart, is derived from the lateral plate mesoderm. Heart development begins with the formation of the cardiac crescent, a group of progenitor cells that differentiate into various cell types, including cardiomyocytes, the muscle cells of the heart. The transcription factor Nkx2.5 plays a critical role in cardiac progenitor specification and its expression is regulated by signaling pathways such as BMP and FGF.
Cardiomyocytes differentiate from progenitor cells and begin to form the cardiac tube, a structure that later undergoes looping and septation to form the four-chambered heart. Unlike skeletal muscle fibers, which are multinucleated, cardiomyocytes are typically mononucleated and interconnected by specialized junctions called intercalated discs that allow synchronized contraction.
Rhythmic contraction of the heart is regulated by the sinoatrial node, which acts as the heart's natural pacemaker. The development of this node and other cardiac structures is tightly controlled by a network of transcription factors, including Nkx2.5, Tbx5, and GATA4. Disruptions in these regulatory networks can lead to congenital heart defects, underscoring the importance of precise control of cardiac muscle development.
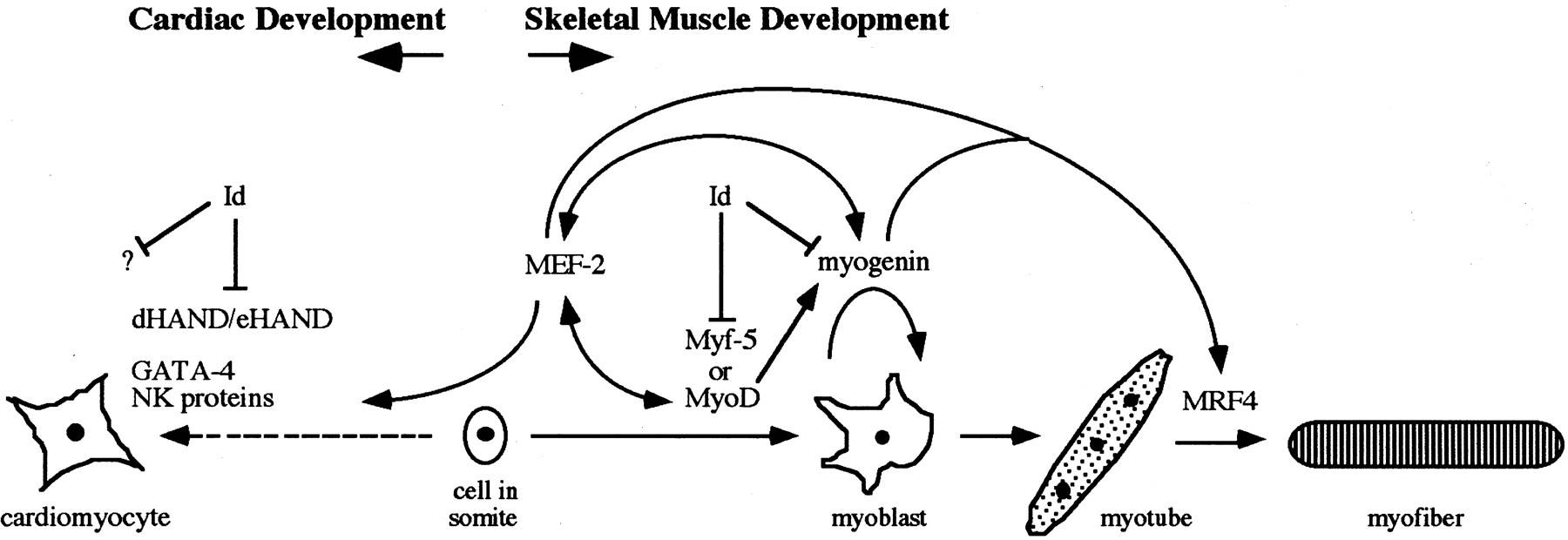
Smooth Muscle Development
Smooth muscle is found in the walls of several internal organs, including blood vessels, the gastrointestinal tract, and the respiratory system. Like cardiac muscle, smooth muscle is derived from the lateral plate mesoderm, but follows a distinct developmental pathway.
Smooth muscle development is regulated by transcription factors such as serum response factor (SRF) and MyoD, which control the expression of genes involved in smooth muscle contraction. Unlike skeletal muscle, smooth muscle fibers are not striated and their contraction is controlled by the autonomic nervous system rather than by voluntary control.
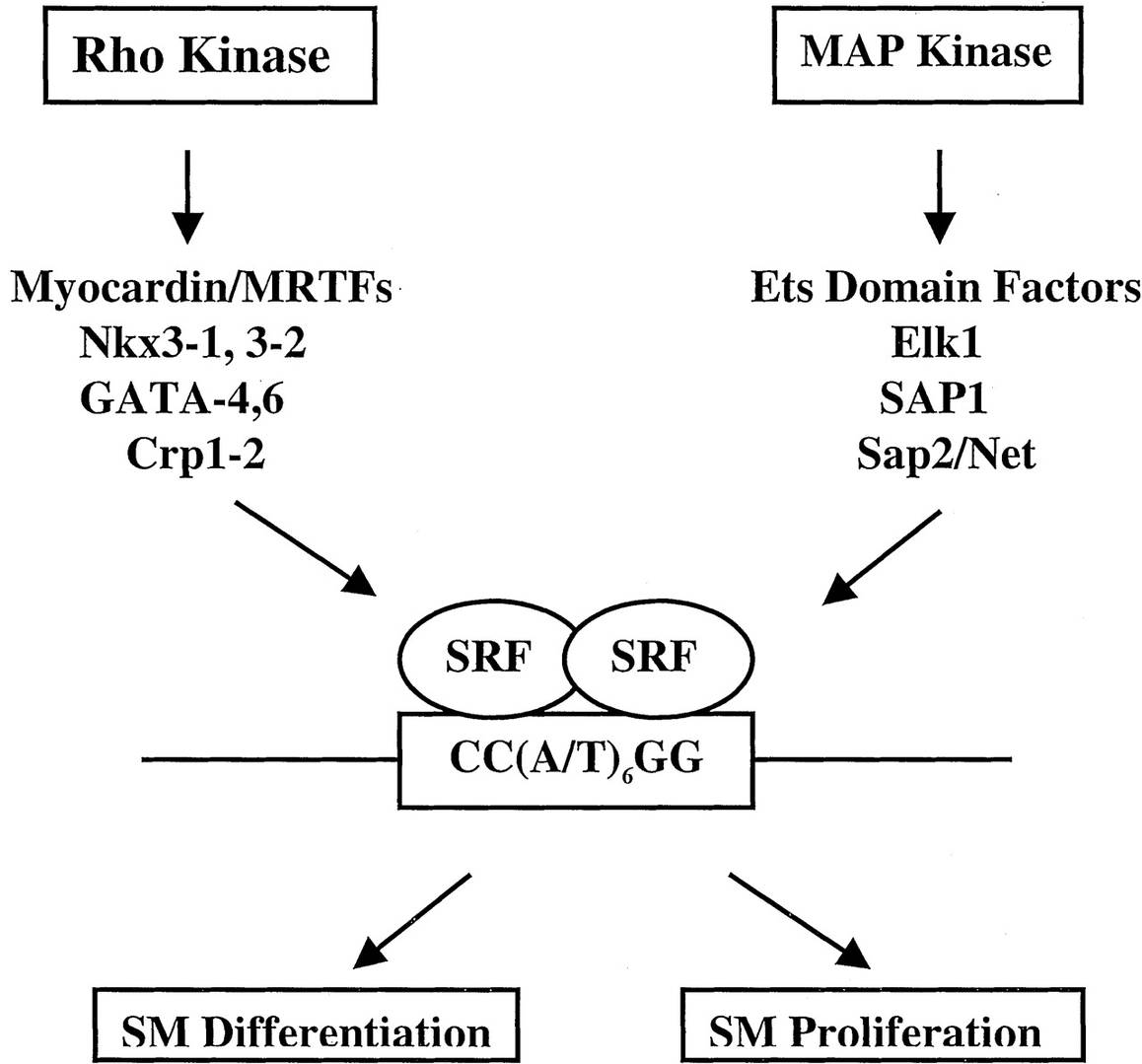
The differentiation of smooth muscle precursors, known as myofibroblasts, is influenced by mechanical signals from the environment and growth factors such as transforming growth factor-beta (TGF-β). Myofibroblasts express smooth muscle actin and other contractile proteins, allowing them to contract in response to physiological stimuli.
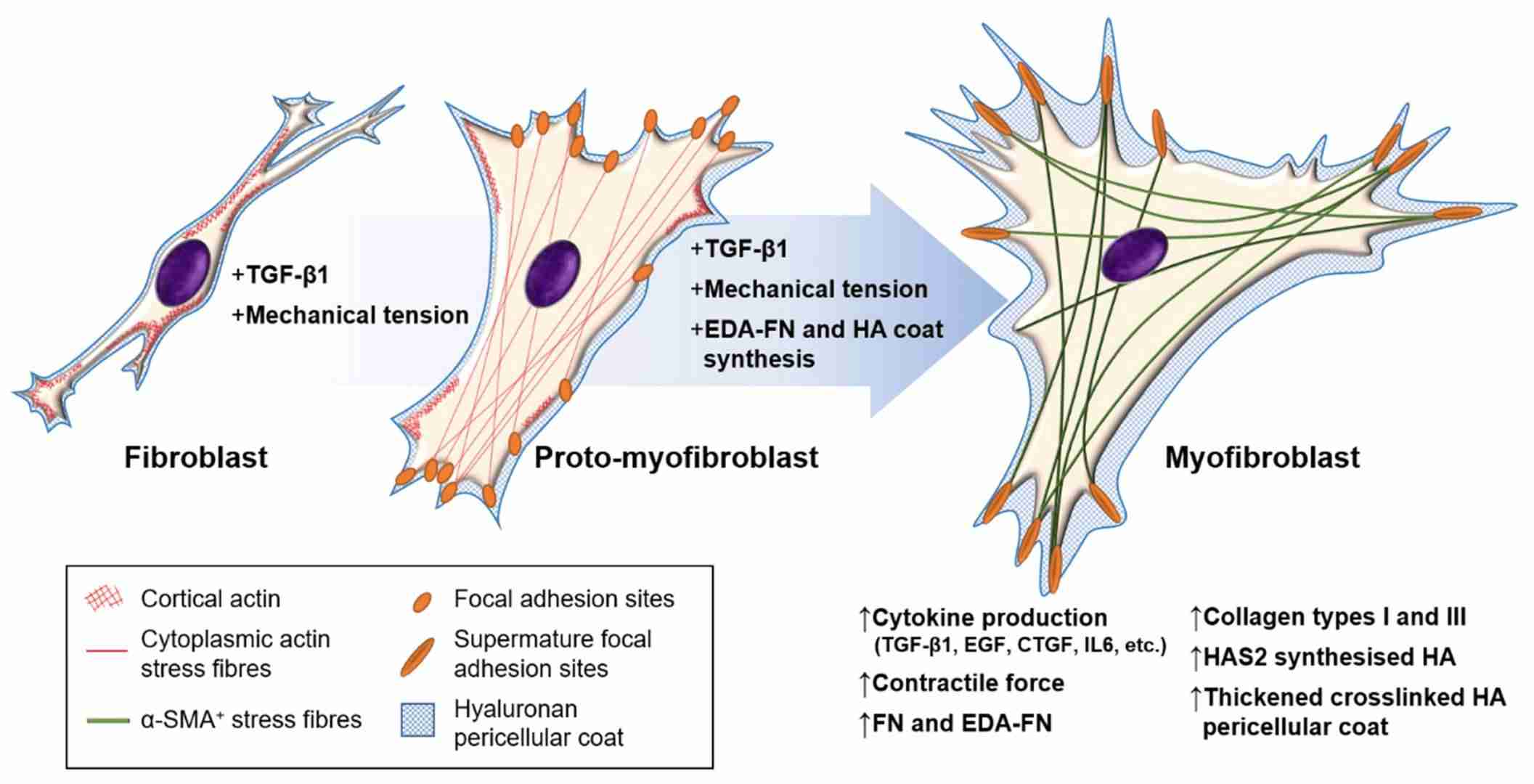
Clinical Implications of Muscle Development
Understanding the mechanisms of muscle development has important implications for medical research and clinical practice. Many inherited diseases, such as muscular dystrophies and congenital myopathies, result from mutations in genes involved in myogenesis. For example, Duchenne muscular dystrophy (DMD) is caused by mutations in the dystrophin gene, resulting in progressive muscle degeneration.
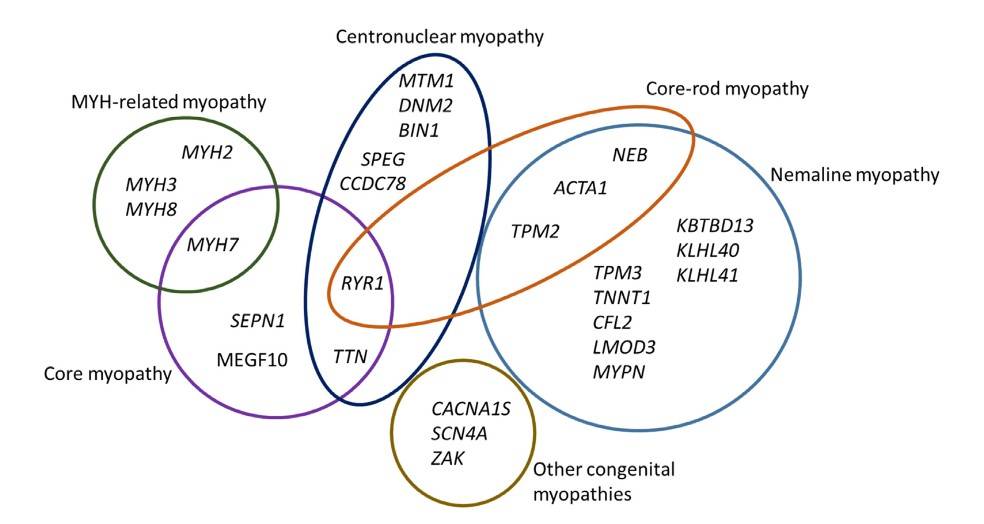
In addition to genetic disorders, research on muscle development has implications for regenerative medicine. The ability to manipulate stem cells to differentiate into muscle cells holds promise for the treatment of muscle injuries and degenerative diseases. For example, researchers are investigating the use of satellite cells, a type of muscle stem cell, to regenerate damaged skeletal muscle tissue.
Cardiac muscle regeneration is another area of interest, particularly in the context of heart disease. Following a heart attack, the heart's ability to regenerate lost cardiomyocytes is limited, leading to permanent damage. Understanding the molecular mechanisms that control cardiac muscle development may provide insights into how to stimulate the regeneration of heart tissue and improve outcomes for patients with heart disease.
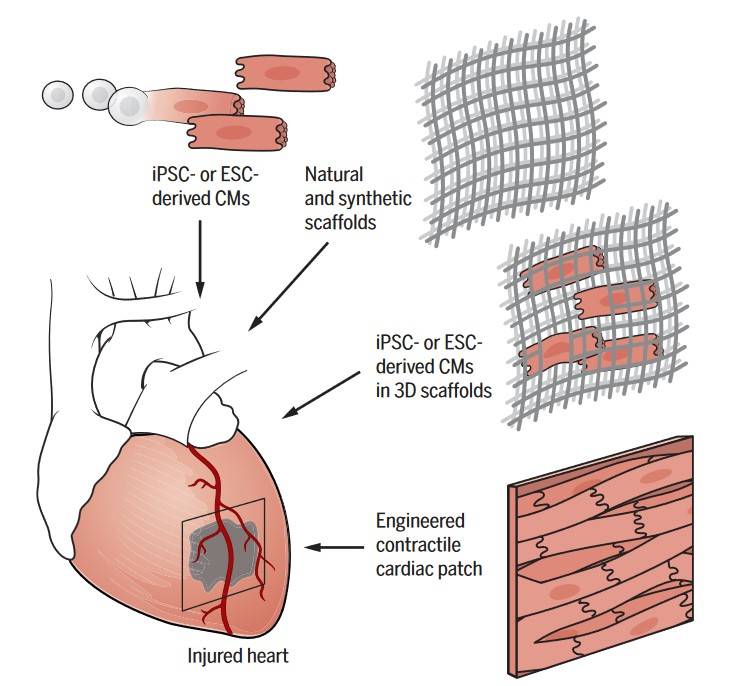
In summary, the development of the muscular system is a highly regulated process involving the interaction of multiple signaling pathways, transcription factors, and cellular processes. From the formation of skeletal muscle fibers to the specialization of cardiac and smooth muscle, myogenesis is critical for the proper function of the body. Understanding these developmental processes not only sheds light on the complexity of embryogenesis but also has important clinical implications for the treatment of muscle-related diseases and injuries. As research into muscle development continues, new insights into the molecular mechanisms that control myogenesis are likely to lead to new therapeutic approaches for regenerating damaged muscle tissue and treating genetic muscle disorders.
Case Study
Case 1: Conerly, M. L.; et al. Distinct activities of Myf5 and MyoD indicate separate roles in skeletal muscle lineage specification and differentiation. Developmental Cell. 2016, 36(4), 375–385.
The transcription factors Myf5 and MyoD, both members of the bHLH family, play critical but distinct roles in skeletal muscle development. Although they bind to the same genomic sites, Myf5 initiates muscle lineage specification by promoting histone acetylation without recruiting RNA polymerase II or significantly activating gene transcription. In contrast, MyoD promotes histone acetylation, recruits Pol II, and robustly activates gene transcription to drive full transcription of the skeletal muscle program. This sequential action highlights how Myf5 and MyoD cooperatively but uniquely regulate different stages of muscle gene activation at shared binding sites.
MyoD and Myf5 both induce chromatin remodeling and histone H4 acetylation (H4Ac) at their binding sites, which supports gene activation. Experiments using transduced fibroblasts show that MyoD and Myf5 similarly increase H4Ac at targeted sites, even though Myf5 itself does not strongly drive transcription. Control analyses confirmed that unrelated genomic E-boxes, which are inaccessible due to nucleosome blocking, exhibit minimal H4Ac in response to either factor, demonstrating the site-specific action of MyoD and Myf5. This suggests that Myf5 and MyoD regulate chromatin accessibility similarly, though with differing effects on gene transcription.
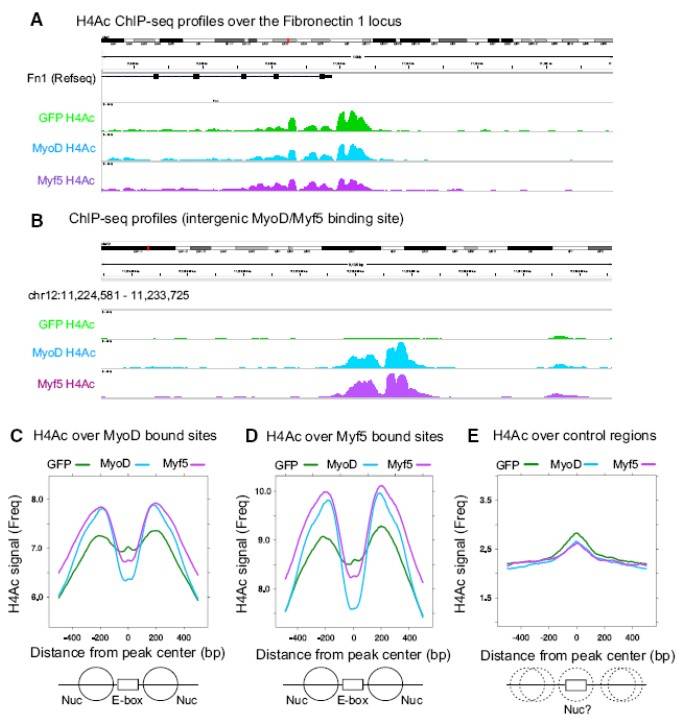
Case 2: Von Maltzahn, J.; et al. Pax7 is critical for the normal function of satellite cells in adult skeletal muscle. Proceedings of the National Academy of Sciences. 2013, 110(41), 16474–16479.
Research on Pax7 in mice shows that this gene is critical for maintaining satellite cell function in adult skeletal muscle. Although an earlier study suggested Pax7 might not be necessary for satellite cells post three weeks of age, new findings reveal that Pax7 deletion leads to satellite cell cycle arrest and disrupted regulation of myogenic factors, preventing effective muscle regeneration. Continuous Pax7 deletion using tamoxifen-induced inactivation results in a significant loss of Pax7-expressing satellite cells and severe muscle atrophy, similar to the effects of direct satellite cell ablation. This highlights Pax7's essential role in both neonatal and adult muscle regeneration and satellite cell proliferation.
In studies of Pax7-deficient satellite cells, researchers induced Pax7 deletion in adult mice using tamoxifen and observed muscle regeneration after injury. In Pax7-deleted mice, muscle regeneration was impaired, with increased interstitial cell accumulation and ectopic fat formation following injury. Continuous tamoxifen treatment further emphasized Pax7's role; Pax7-deficient mice displayed severe regeneration deficits, including fewer regenerating fibers and extensive fat, fibrosis, and calcium deposits. Re-injury exacerbated these effects, confirming that Pax7 is critical for satellite cell function and effective muscle regeneration in adult skeletal muscle.
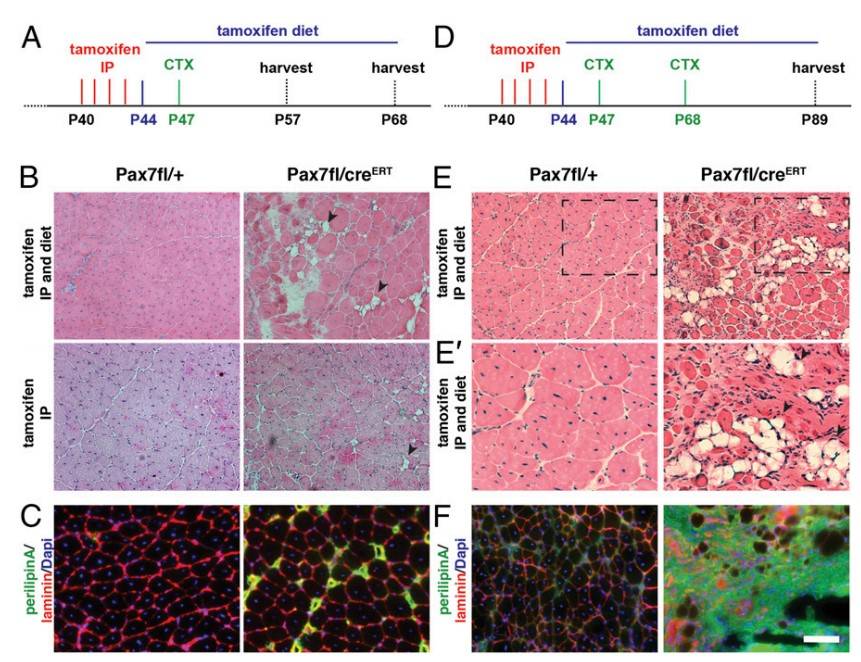
References
- Conerly, M. L., Yao, Z., Zhong, J. W., Groudine, M., & Tapscott, S. J. (2016). Distinct activities of myf5 and MyoD indicate separate roles in skeletal muscle lineage specification and differentiation. Developmental Cell, 36(4), 375–385.
- Mably, J. D., & Liew, C.-C. (1996). Factors involved in cardiogenesis and the regulation of cardiac-specific gene expression. Circulation Research, 79(1), 4–13.
- Majesky, M. W. (2003). Decisions, decisions … SRF coactivators and smooth muscle myogenesis. Circulation Research, 92(8), 824–826.
- Nassari, S. (2017). The contribution of connective tissues to muscle morphogenesis: An unexpected role for CXCL12 and CXCL14 chemokines. Phdthesis, Université Pierre et Marie Curie - Paris VI; Freie Universität (Berlin).
- Pelin, K., & Wallgren-Pettersson, C. (2019). Update on the genetics of congenital myopathies. Seminars in Pediatric Neurology, 29, 12–22.
- Tai, Y., Woods, E. L., Dally, J., Kong, D., Steadman, R., Moseley, R., & Midgley, A. C. (2021). Myofibroblasts: Function, formation, and scope of molecular therapies for skin fibrosis. Biomolecules, 11(8), 1095.
- Tani, S., Chung, U., Ohba, S., & Hojo, H. (2020). Understanding paraxial mesoderm development and sclerotome specification for skeletal repair. Experimental & Molecular Medicine, 52(8), 1166–1177.
- Tzahor, E., & Poss, K. D. (2017). Cardiac regeneration strategies: Staying young at heart. Science, 356(6342), 1035–1039.
- Von Maltzahn, J., Jones, A. E., Parks, R. J., & Rudnicki, M. A. (2013). Pax7 is critical for the normal function of satellite cells in adult skeletal muscle. Proceedings of the National Academy of Sciences, 110(41), 16474–16479.