Signal Transduction in Cancer
Creative BioMart Signal Transduction in Cancer Product List
Immunology Background
Background
Signal transduction refers to the processes by which cells respond to external stimuli and convey messages through a network of molecular events that lead to specific functional outcomes such as proliferation, differentiation, migration, or apoptosis. Under normal physiological conditions, these pathways are tightly regulated to ensure that cellular responses are appropriate. However, aberrations in these pathways can lead to uncontrolled cellular processes, one of the hallmarks of cancer. In cancer, mutations in key signaling molecules or dysregulation of their associated pathways drive tumor development, growth and metastasis.
Key Signaling Pathways in Cancer
Receptor Tyrosine Kinases (RTKs)
Receptor tyrosine kinases (RTKs) are a class of membrane receptors that, upon ligand binding, activate a range of downstream signaling pathways, including the MAPK (mitogen-activated protein kinase), PI3K/AKT (phosphoinositide 3-kinase), and JAK/STAT (Janus kinase/signal transducer and activator of transcription) pathways. RTKs regulate processes such as cell proliferation, survival, and differentiation. However, in cancer, mutations and overexpression of RTKs result in aberrant signaling and tumor growth.
- EGFR (Epidermal Growth Factor Receptor): Mutations or amplification of EGFR are frequently found in cancers, such as non-small cell lung cancer (NSCLC). These aberrations lead to uncontrolled activation of downstream signaling pathways, including MAPK and PI3K/AKT, driving uncontrolled proliferation and resistance to apoptosis. Targeted therapies, such as tyrosine kinase inhibitors (TKIs), have been developed to block aberrant EGFR signaling.
- HER2 (Human Epidermal Growth Factor Receptor 2): Overexpression of HER2 is a common feature of certain breast cancers. HER2-mediated signaling promotes cell proliferation and survival, contributing to aggressive tumor growth. HER2-targeted therapies, such as trastuzumab, are used to interfere with this signaling.
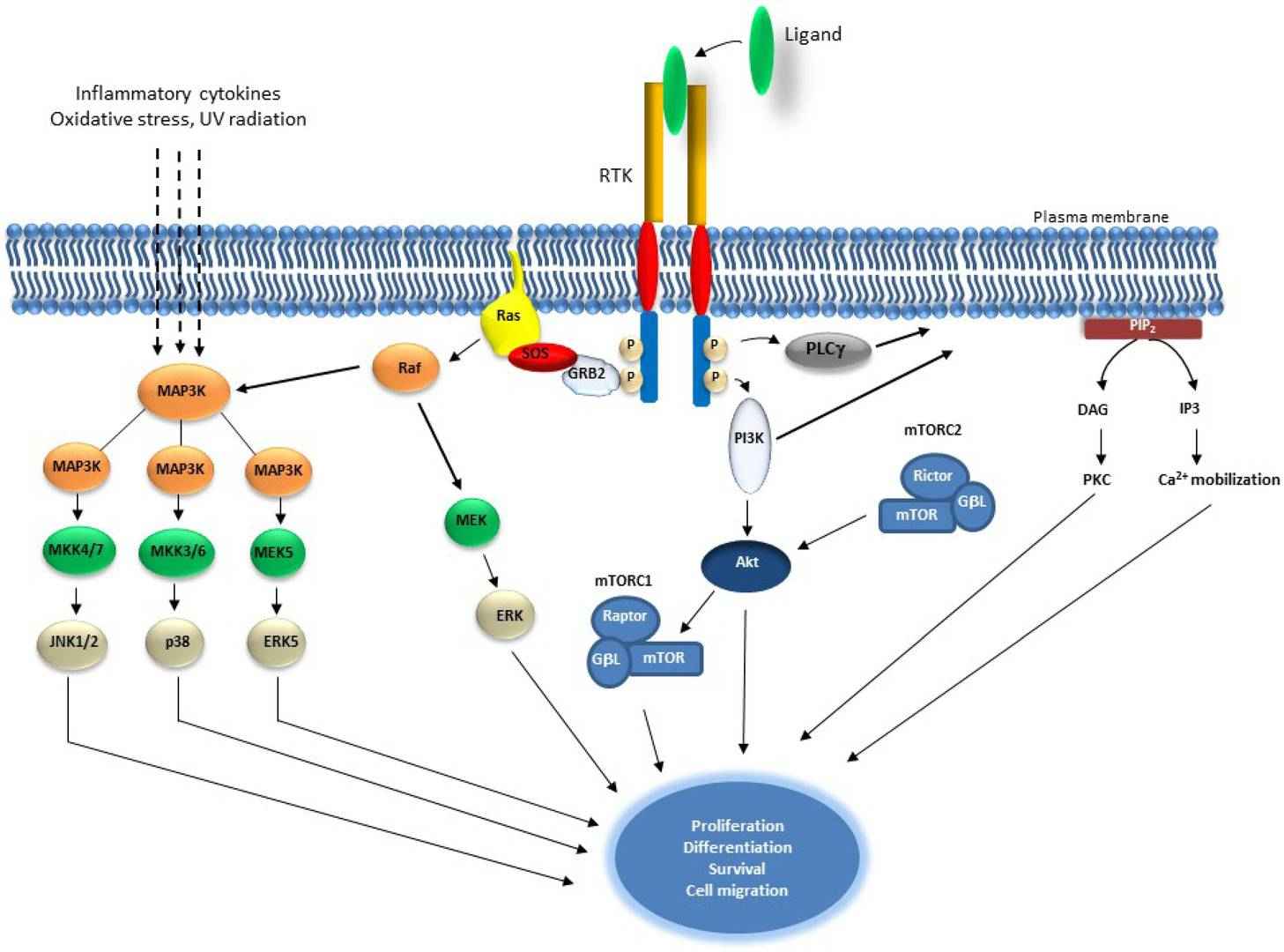
MAPK Pathway
The MAPK pathway is a critical regulator of cell division, differentiation, and survival. It is initiated by the binding of growth factors to RTKs, which subsequently activates a cascade of kinases, including RAS, RAF, MEK, and ERK. In cancer, mutations in components of the MAPK pathway often result in constitutive activation.
- RAS: Mutations in the RAS family of oncogenes (KRAS, NRAS, HRAS) are among the most common mutations in human cancers, particularly in pancreatic, colorectal, and lung cancers. Mutant RAS is constitutively active, resulting in constant stimulation of downstream pathways such as MAPK and PI3K/AKT, which promotes cell proliferation and survival.
- BRAF: BRAF mutations are common in melanoma, the most common being the V600E mutation. This mutation leads to constitutive activation of the MAPK pathway, which promotes tumorigenesis. BRAF inhibitors, such as vemurafenib, have been developed to target this mutation.
PI3K/AKT/mTOR Pathway
The PI3K/AKT/mTOR pathway regulates cell growth, proliferation, and survival by responding to signals from growth factors and nutrients. Dysregulation of this pathway is a common feature in many cancers, including breast, lung, and ovarian cancers.
- PI3K: PI3K mutations or amplifications lead to continuous activation of downstream signaling, including the phosphorylation of AKT, a key regulator of cell survival. PTEN, a tumor suppressor, negatively regulates the PI3K pathway by dephosphorylating PIP3 (phosphatidylinositol (3,4,5)-trisphosphate). Loss or mutation of PTEN results in hyperactivation of the PI3K pathway, leading to uncontrolled cell growth.
- AKT: AKT phosphorylates various downstream targets that regulate cell cycle progression, metabolism, and apoptosis. In cancer, hyperactivated AKT prevents apoptosis and promotes cell survival, contributing to tumor growth.
- mTOR (Mammalian Target of Rapamycin): mTOR is a downstream effector of the PI3K/AKT pathway and regulates protein synthesis and cell growth. mTOR is often hyperactivated in cancer due to upstream mutations in PI3K, AKT, or loss of PTEN. Inhibitors of mTOR, such as rapamycin and its analogs, have been developed to target this pathway in cancer therapy.
Wnt/β-catenin Pathway
The Wnt/β-catenin pathway plays a critical role in the regulation of cell fate, proliferation and migration. Under normal conditions, β-catenin levels are tightly regulated by a destruction complex that includes the tumor suppressor APC (adenomatous polyposis coli). In the presence of Wnt signals, this destruction complex is inhibited, allowing β-catenin to accumulate and translocate to the nucleus, where it activates the transcription of target genes involved in cell proliferation.
- Mutations in APC: In colorectal cancers, mutations in APC lead to the inability of the destruction complex to degrade β-catenin, resulting in its accumulation and constant activation of Wnt signaling. This promotes uncontrolled cell proliferation and tumor growth.
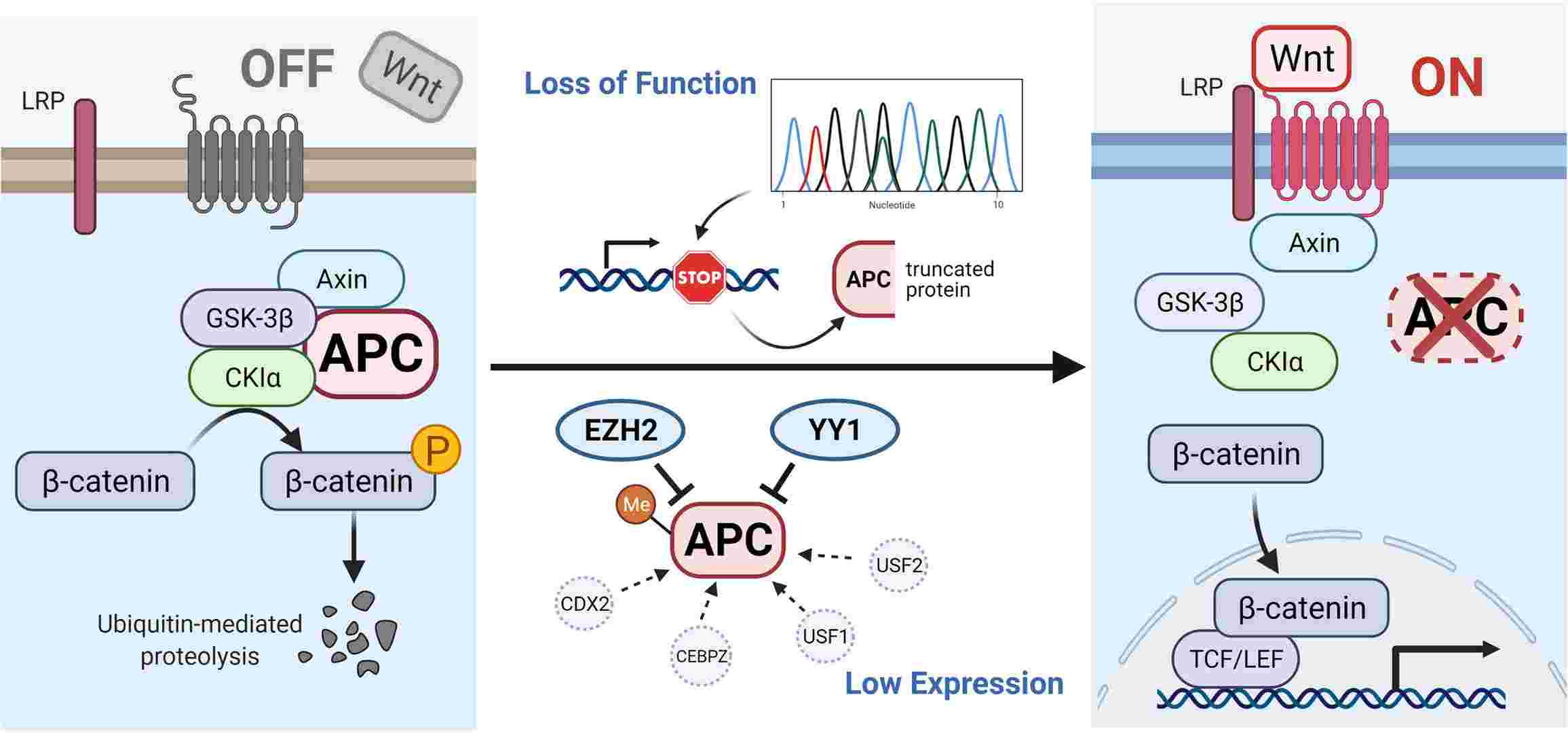
- Mutations in β-catenin: Mutations that stabilize β-catenin can also contribute to aberrant activation of the Wnt pathway, as observed in cancers such as hepatocellular carcinoma.
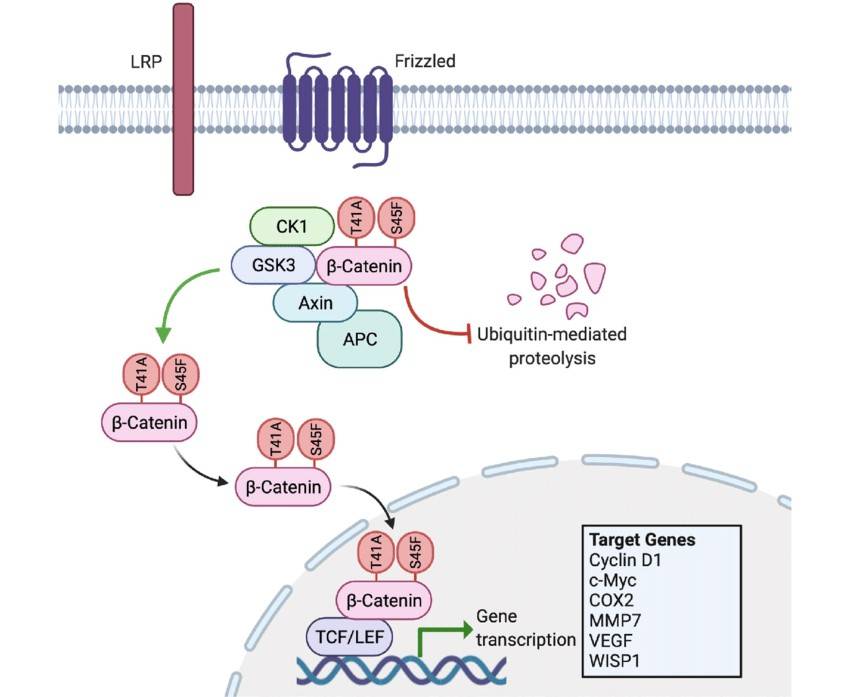
JAK/STAT Pathway
The JAK/STAT pathway is activated by cytokines and growth factors and regulates immune responses, hematopoiesis and cell growth. Aberrations in this pathway are common in hematologic malignancies and some solid tumors.
- Constitutive STAT Activation: In cancer, dysregulation of JAK/STAT signaling often results in the constitutive activation of STAT proteins (especially STAT3 and STAT5). Activated STATs translocate to the nucleus, where they induce the expression of genes involved in cell proliferation, survival, and immune evasion. Inhibition of this pathway is an area of interest in cancer therapy, with various JAK inhibitors currently being developed.
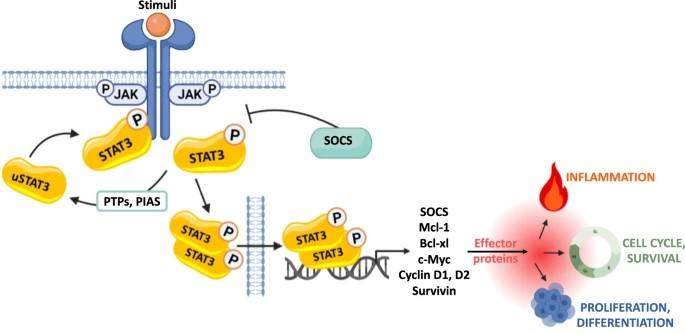
Hedgehog Signaling Pathway
The Hedgehog (Hh) signaling pathway is essential for embryonic development, but its aberrant activation has been implicated in several cancers, including basal cell carcinoma and medulloblastoma. In the absence of Hedgehog ligands, the pathway is inactive and the transcription factor GLI is degraded. When Hedgehog ligands bind to the Patched (PTCH) receptor, the repression of Smoothened (SMO) is relieved, leading to activation of GLI and transcription of target genes.
- SMO Mutations: Mutations in SMO or PTCH can lead to ligand-independent activation of Hedgehog signaling and promote tumor growth. SMO inhibitors, such as vismodegib, have been developed for the treatment of Hedgehog pathway-dependent cancers.
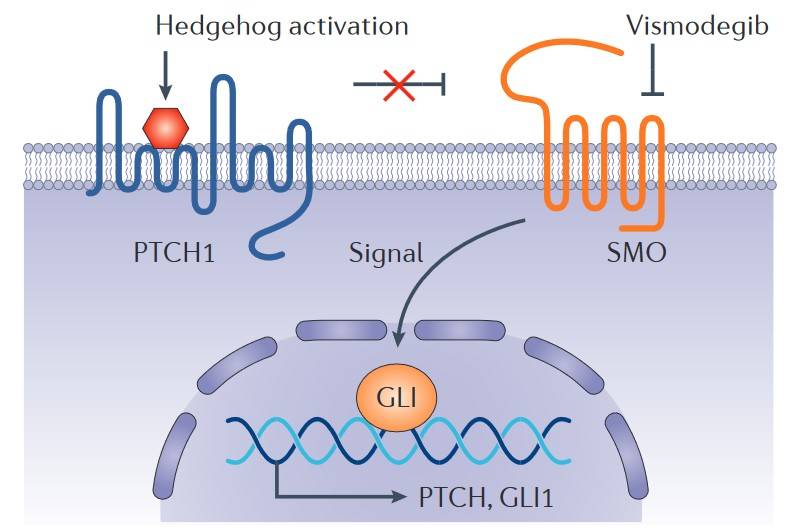
FAK-Src Signaling
FAK (Focal Adhesion Kinase) and Src are non-receptor tyrosine kinases involved in signaling pathways that regulate cell adhesion, migration, proliferation, and survival. In cancer, the FAK-Src signaling axis is often hyperactivated, contributing to tumor growth, invasion, and metastasis.
- FAK Overexpression: FAK is frequently overexpressed in various cancers, including breast, colon and lung cancer. Its overactivity leads to increased cell motility and invasion by promoting integrin signaling, which alters cell-extracellular matrix (ECM) interactions. FAK signaling is critical for epithelial-to-mesenchymal transition (EMT), a process that facilitates cancer metastasis.
- Src Activation: Src enhances the phosphorylation of FAK and other proteins, activating downstream pathways such as MAPK and PI3K/AKT. This signaling promotes cancer cell survival and proliferation, while Src-driven adhesion dynamics promote tumor cell metastasis. Targeting FAK-Src signaling has become a therapeutic strategy to block cancer cell migration and invasion.
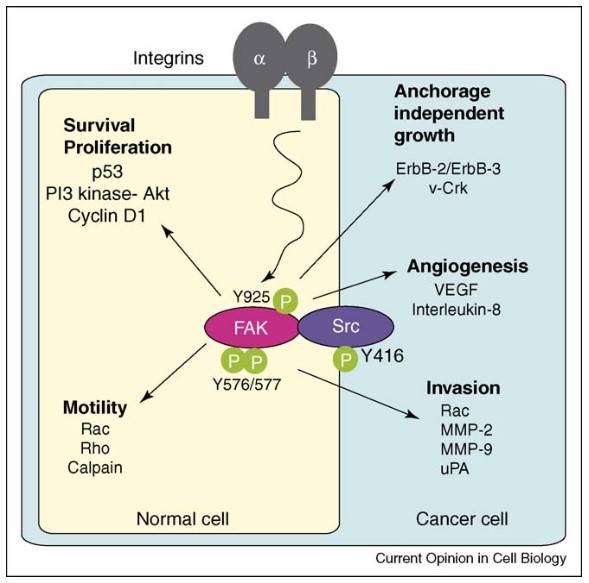
Ubiquitin-Proteasome Pathway
The ubiquitin-proteasome system (UPS) is essential for protein degradation and turnover, regulating various cellular processes such as cell cycle control, apoptosis and DNA repair. Dysregulation of this pathway plays an important role in cancer by affecting the stability of oncogenes and tumor suppressors.
- Oncoprotein Stabilization: Many oncogenes, such as Myc and cyclin D1, are regulated by the ubiquitin-proteasome system. Impaired degradation of these proteins leads to their accumulation, driving uncontrolled cell proliferation. For example, aberrant proteasome activity can prevent the degradation of β-catenin in the Wnt signaling pathway, promoting tumorigenesis.
- Tumor Suppressor Degradation: The UPS is also responsible for the degradation of tumor suppressor proteins such as p53. In many cancers, overexpression of E3 ubiquitin ligases (such as MDM2) leads to increased degradation of p53, preventing its tumor suppressor functions such as apoptosis induction and cell cycle arrest.
- Proteasome Inhibitors in Cancer Therapy: Targeting the ubiquitin-proteasome system has proven to be an effective cancer therapy. Proteasome inhibitors such as bortezomib are used to treat multiple myeloma and other cancers. These drugs block the degradation of pro-apoptotic factors, leading to the accumulation of proteins that promote cancer cell death.
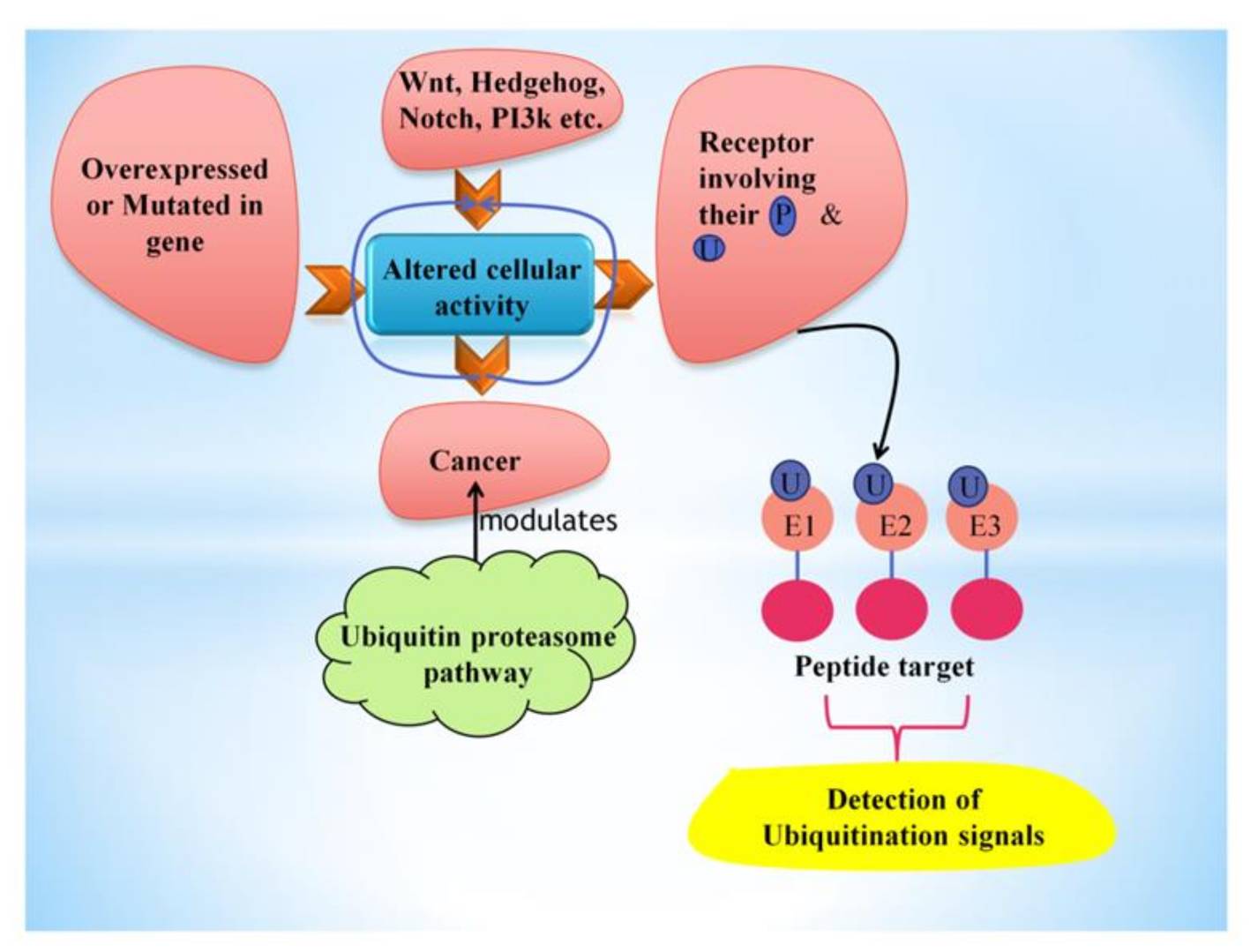
Impact on Cancer Development
Uncontrolled Proliferation
Aberrant signaling in cancer cells often results in uncontrolled proliferation. Pathways such as MAPK, PI3K/AKT, and Wnt are primarily involved in promoting cell division and survival. Mutations in key signaling proteins, such as RAS, BRAF, and PI3K, allow cancer cells to bypass normal regulatory mechanisms, enabling continuous cell growth even in the absence of external growth signals.
Resistance to Apoptosis
Apoptosis, or programmed cell death, is a critical mechanism for eliminating damaged or abnormal cells. Cancer cells often develop resistance to apoptosis through the dysregulation of signaling pathways that control cell survival. For example, activation of the PI3K/AKT pathway promotes the phosphorylation and inactivation of pro-apoptotic factors such as BAD and FOXO (FOXO1 and FOXO4), preventing apoptosis and allowing cancer cells to survive under adverse conditions.
Angiogenesis
Angiogenesis, the formation of new blood vessels, is necessary for tumor growth and metastasis. Cancer cells activate signaling pathways, such as VEGF (vascular endothelial growth factor) signaling, to promote angiogenesis. VEGF is a key player in this process, and its expression is often upregulated in response to hypoxia or activation of oncogenic signaling pathways, such as PI3K/AKT and MAPK. Targeting VEGF with therapies like bevacizumab can inhibit angiogenesis and slow tumor growth.
Metastasis
The metastatic spread of cancer cells to distant organs involves changes in cell adhesion, migration, and invasion. Signaling pathways, such as Wnt/β-catenin and PI3K/AKT, regulate the expression of genes involved in epithelial-mesenchymal transition (EMT), a process that allows cancer cells to become more migratory and invasive. Additionally, activation of the Hedgehog pathway has been implicated in promoting metastasis in certain cancers.
Immune Evasion
Cancer cells can evade immune surveillance by exploiting signaling pathways involved in immune regulation. For example, the activation of the JAK/STAT pathway can upregulate the expression of PD-L1 (programmed death-ligand 1), an immune checkpoint molecule that inhibits T-cell-mediated immune responses. Immune checkpoint inhibitors, such as anti-PD-1 and anti-PD-L1 therapies, have been developed to restore immune system activity against cancer cells.
Therapeutic Implications
The understanding of signal transduction pathways in cancer has led to the development of targeted therapies designed to inhibit specific molecules within these pathways. Some notable examples include:
- Tyrosine Kinase Inhibitors (TKIs): TKIs, such as gefitinib and erlotinib, target aberrant RTK signaling, specifically EGFR mutations, in lung cancer.
- PI3K/AKT/mTOR Inhibitors: Drugs targeting PI3K, AKT, and mTOR, such as everolimus and alpelisib, have been developed to inhibit the hyperactive PI3K/AKT/mTOR pathway in cancers with PTEN mutations or PI3K amplifications.
- MEK Inhibitors: MEK inhibitors, such as trametinib, are used to block the MAPK pathway in cancers with BRAF mutations, particularly melanoma.
- Immunotherapies: Targeting immune checkpoint molecules like PD-1 and PD-L1 has revolutionized cancer treatment, particularly in cancers that exploit the JAK/STAT pathway for immune evasion.
In summary, signal transduction pathways play a critical role in maintaining normal cellular function. However, their dysregulation is a key driver of cancer development and progression. Understanding the molecular mechanisms behind these pathways has led to significant advances in targeted cancer therapies and improved patient outcomes. Despite these advances, challenges remain in overcoming drug resistance and developing therapies that target multiple pathways simultaneously. Continued research into cancer signaling holds the promise of more effective and personalized cancer treatments in the future.
Case Study
Case 1: Fennell, L. J.; et al. APC mutation marks an aggressive subtype of BRAF mutant colorectal cancers. Cancers.2012, 12(5), 1171.
WNT activation is a key feature of colorectal cancer, with BRAF mutations present in 15% of cases. However, the role of mutations in WNT signaling regulators in this context remains unclear. Researchers analyzed the mutational landscape of WNT signaling regulators in BRAF mutant cancers using exome sequencing of 24 BRAF mutant colorectal cancers and data from 175 publicly available exomes. They examined somatic mutations and potential driver mutations in WNT signaling. The effects of APC and BRAF mutations were studied in in vivo models using APCmin/+ and BRAFV637/Villin-CreERT2/+ mice.
The findings revealed that RNF43 was the most frequently mutated WNT regulator, with mutations in the beta-catenin destruction complex occurring in 48% of cases. Potential cancer drivers in WNT signaling, including MEN1, GNG12, and WNT16, were identified through hotspot analysis. Truncating APC mutations were found in 20.8% of cancers. Overall, WNT signaling is commonly mutated in BRAF mutant colorectal cancers, with WNT16 and MEN1 potentially acting as novel drivers of aberrant signaling. Co-mutations of BRAF and APC lead to an aggressive cancer phenotype and poor patient outcomes.
The researchers examined the mutational profile of WNT signaling genes in 512 additional colorectal cancers from the Cancer Genome Atlas that were BRAF wild type. The most frequently mutated genes are significantly different in BRAF wild type cancers. APC was mutated in 82% of these cancers, compared to only 28% of BRAF mutant cancers.
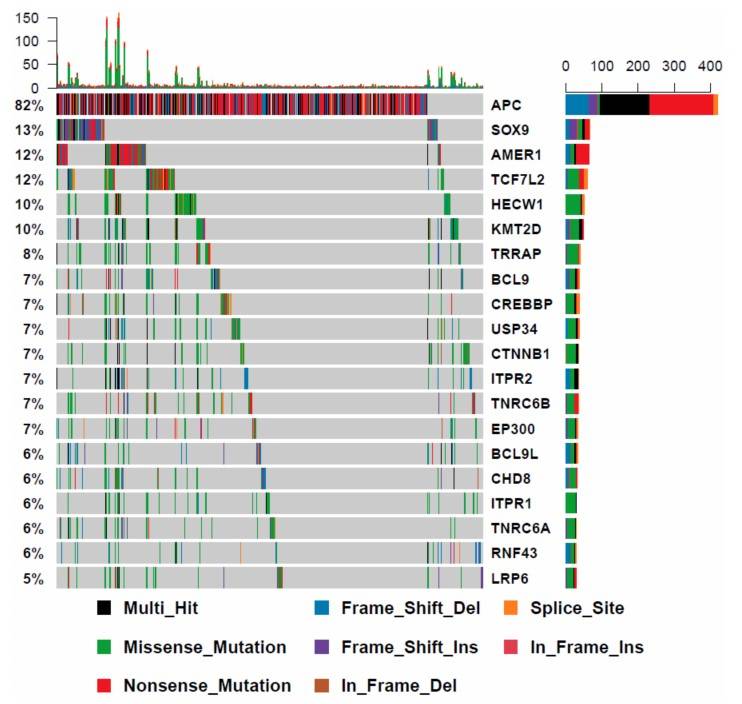
Case 2: Sandilands, E.; et al. Autophagic targeting of Src promotes cancer cell survival following reduced FAK signalling. Nature Cell Biology. 2012, 14(1), 51–60.
This study uncovers a mechanism that cancer cells use to survive when the Src/FAK pathway is severely disrupted. When FAK is depleted, FAK-proficient cells are detached, or non-phosphorylatable FAK proteins are expressed, active Src is sequestered away from focal adhesions into intracellular puncta that co-localize with autophagy regulators. Inhibition of autophagy returns active Src to focal adhesions, resulting in cancer cell death. This autophagic targeting of active Src involves a Src-LC3B complex mediated by c-Cbl through its LC3 interacting region, independent of c-Cbl E3 ligase activity. This process helps cancer cells survive when Src/FAK signaling is impaired, revealing a vulnerability that could be exploited therapeutically.
Active Src colocalized with c-Cbl at focal adhesions in FAK+/+ cells (Fig. 9a, left, solid arrows) and in intracellular puncta in FAK-/- cells (Fig. 9a, middle panel, dashed arrows). Treatment with chloroquine enhanced the accumulation of active Src in c-Cbl-containing puncta (Fig. 9a, right, dashed arrows), and immunoprecipitation of endogenous c-Cbl revealed an increase in binding to active Src in the absence of FAK (Fig. 9b). Next, c-Cbl expression was knocked down (Fig. 9c). In FAK-/- cells, c-Cbl siRNA restored active Src to peripheral adhesions (Fig. 9d, bottom right, solid arrows) and significantly reduced LC3B binding to Src (Fig. 9e), suggesting that c-Cbl mediates the formation of the complex between Src and LC3B, thereby promoting selective autophagic targeting. Thus, proteins involved in selective autophagic targeting of active Src, namely c-Cbl, active Src and LC3B, are all present at focal adhesions in FAK+/+ cancer cells. Upon perturbation of flux through the Src/FAK pathway, active Src forms a complex with LC3B and is selectively targeted to autophagosomes in a c-Cbl-dependent manner.
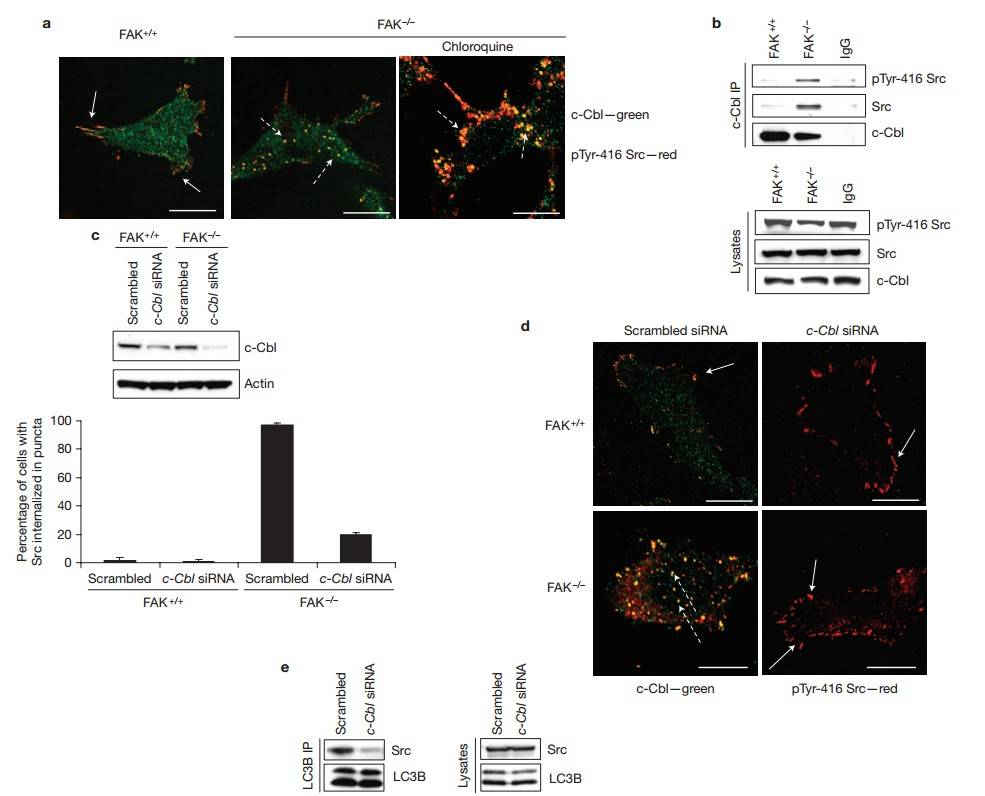
References
- Dlugosz, A., Agrawal, S., & Kirkpatrick, P. (2012). Vismodegib. Nature Reviews Drug Discovery, 11(6), 437–438.
- Fennell, L. J., Kane, A., Liu, C., McKeone, D., Fernando, W., Su, C., Bond, C., Jamieson, S., Dumenil, T., Patch, A.-M., Kazakoff, S. H., Pearson, J. V., Waddell, N., Leggett, B., & Whitehall, V. L. J. (2020). APC mutation marks an aggressive subtype of BRAF mutant colorectal cancers. Cancers, 12(5), 1171.
- Fu, D., Hu, Z., Xu, X., Dai, X., & Liu, Z. (2022). Key signal transduction pathways and crosstalk in cancer: Biological and therapeutic opportunities. Translational Oncology, 26, 101510.
- Lei, Z.-N., Teng, Q.-X., Tian, Q., Chen, W., Xie, Y., Wu, K., Zeng, Q., Zeng, L., Pan, Y., Chen, Z.-S., & He, Y. (2022). Signaling pathways and therapeutic interventions in gastric cancer. Signal Transduction and Targeted Therapy, 7(1), 1–38.
- McLean, T. D., Duchi, S., & Di Bella, C. (2022). Molecular pathogenesis of sporadic desmoid tumours and its implications for novel therapies: A systematised narrative review. Targeted Oncology, 17(3), 223–252.
- Mitra, S. K., & Schlaepfer, D. D. (2006). Integrin-regulated FAK–Src signaling in normal and cancer cells. Current Opinion in Cell Biology, 18(5), 516–523.
- Regad, T. (2015). Targeting RTK signaling pathways in cancer. Cancers, 7(3), 1758–1784.
- Sandilands, E., Serrels, B., McEwan, D. G., Morton, J. P., Macagno, J. P., McLeod, K., Stevens, C., Brunton, V. G., Langdon, W. Y., Vidal, M., Sansom, O. J., Dikic, I., Wilkinson, S., & Frame, M. C. (2012). Autophagic targeting of Src promotes cancer cell survival following reduced FAK signalling. Nature Cell Biology, 14(1), 51–60.
- Semenzato, G., Calabretto, G., Teramo, A., Gasparini, V. R., Rampazzo, E., Barilà, G., & Zambello, R. (2024). The constitutive activation of STAT3 gene and its mutations are at the crossroad between LGL leukemia and autoimmune disorders. Blood Cancer Journal, 14(1), 1–8.
- Sever, R., & Brugge, J. S. (2015). Signal transduction in cancer. Cold Spring Harbor Perspectives in Medicine, 5(4), a006098.
- Sharma, A., Khan, H., Singh, T. G., Grewal, A. K., Najda, A., Kawecka-Radomska, M., Kamel, M., Altyar, A. E., & Abdel-Daim, M. M. (2021). Pharmacological modulation of ubiquitin-proteasome pathways in oncogenic signaling. International Journal of Molecular Sciences, 22(21), 11971.
- Zhu, L., Li, X., Yuan, Y., Dong, C., & Yang, M. (2021). APC promoter methylation in gastrointestinal cancer. Frontiers in Oncology, 11, 653222.