Vascular Endothelium
Creative BioMart Vascular Endothelium Product List
- ADAMs on Endothelial Cells
- Adhesion Molecules on Endothelial Cells
- BMPs and Regulators Secreted by Endothelial Cells
- Endothelial Cell Coagulation Molecules
- Endothelial Cell Development Molecules
- Endothelial Cell Markers
- Enzymes Secreted by Endothelial Cells
- GTPases and Regulators in Endothelial Cells
- Immune Regulatory Molecules on Endothelial Cells
- Kinases and Phosphatases in Endothelial Cells
- Neurotransmitter Receptors on Endothelial Cells
- Peptide Receptors on Endothelial Cells
- Transcription Regulators in Endothelial Cells
- Uptake and Transport Molecules on Endothelial Cells
- VEGF Receptors and Regulators on Endothelial Cells
Immunology Background
Background
The vascular endothelium is a monolayer of endothelial cells that line the inner surface of blood vessels. This dynamic tissue plays a critical role in maintaining vascular homeostasis, regulating blood flow, and contributing to various physiological and pathological processes. The endothelium is not merely a passive barrier, but an active participant in the modulation of vascular tone, inflammation, and coagulation.
Structure of the Vascular Endothelium
The vascular endothelium consists mainly of endothelial cells. These cells have different morphological characteristics depending on their location in the vascular tree. In large vessels, endothelial cells are typically polygonal and densely packed. In smaller capillaries, they are more elongated and flattened. The endothelium is anchored to the underlying basement membrane, a thin layer of extracellular matrix. This membrane provides structural support and regulates cell behavior.
The junctions between endothelial cells play a critical role in maintaining barrier function. They are primarily formed by tight junctions and adherens junctions. These junctions control paracellular permeability and are influenced by several signaling molecules, including vascular endothelial growth factor (VEGF) and transforming growth factor-beta (TGF-β).
Endothelial cells can be categorized into different subtypes. These subtypes are based on their anatomical location and functional specialization.
- Arterial Endothelial Cells: These cells line the walls of the arteries that carry oxygenated blood from the heart to tissues throughout the body. Arterial endothelial cells are subjected to high shear stress due to the rapid flow of blood at high pressure. To withstand these forces, they adopt a more elongated shape and align themselves in the direction of blood flow. These cells are particularly sensitive to biomechanical forces and play a key role in regulating blood pressure. They do this by releasing vasodilators such as nitric oxide (NO). Arterial endothelial dysfunction is commonly associated with atherosclerosis. In this condition, inflammation and plaque formation can lead to cardiovascular disease.
- Venous Endothelial Cells: Venous endothelial cells are found in veins, where blood returns to the heart at a lower pressure than in arteries. These cells are more cuboidal in shape and experience less shear stress. As a result, they are structurally and functionally different from arterial endothelial cells. Venous endothelial cells are involved in maintaining vascular permeability, which allows the exchange of fluids and macromolecules between blood and tissues. In contrast to arterial endothelial cells, venous endothelial cells play an important role in thrombosis. They participate in clot formation and interact with blood components such as platelets.
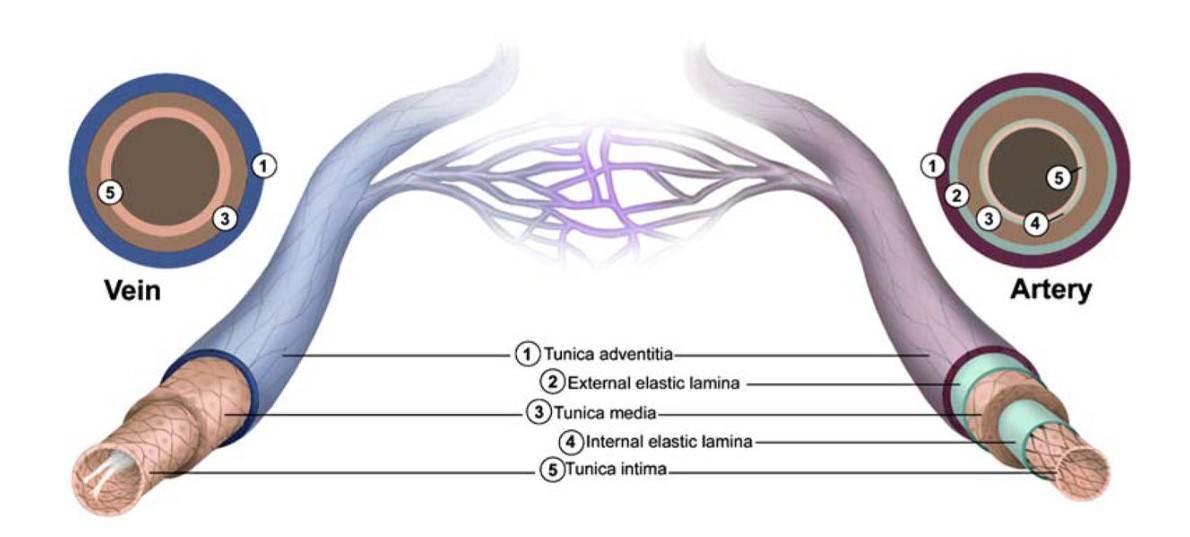
- Capillary Endothelial Cells: Capillary endothelial cells form the lining of capillaries, the smallest blood vessels. These vessels are responsible for nutrient and gas exchange between blood and tissues. Depending on their location and permeability requirements, capillary endothelial cells are classified into three main types: continuous, fenestrated, and discontinuous endothelium. Continuous endothelium is found in the brain and muscles. It has tight junctions that limit permeability. Fenestrated endothelium is found in the kidneys and intestines. It allows greater permeability due to pores (fenestrae) in the cells. Discontinuous or sinusoidal endothelium is found in the liver and spleen. It has large gaps that allow free passage of larger molecules and even cells.
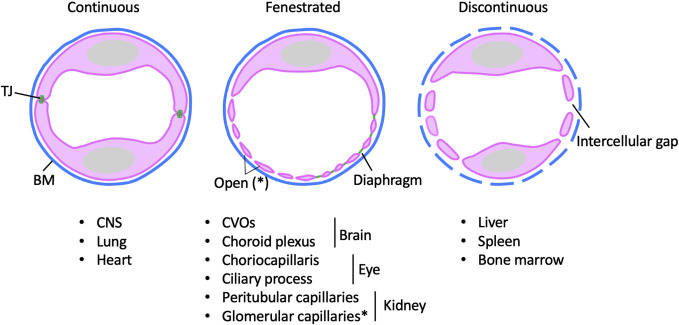
- Lymphatic Endothelial Cells: These cells line the vessels of the lymphatic system. The lymphatic system is responsible for returning interstitial fluid from tissues to the bloodstream. Lymphatic endothelial cells differ structurally from vascular endothelial cells. They play key roles in maintaining fluid balance and immune function. They assist in the absorption of dietary fats and are involved in the transport of immune cells throughout the body. Dysfunction of lymphatic endothelial cells can lead to lymphedema, a condition characterized by fluid accumulation and swelling in tissues.
Functions of the Vascular Endothelium
The vascular endothelium performs several critical functions that are essential for maintaining cardiovascular health.
Regulation of Vascular Tone
Endothelial cells release a variety of signaling molecules that regulate vascular tone, the degree to which blood vessels constrict or dilate. The best known of these is nitric oxide (NO), which is synthesized from L-arginine by endothelial nitric oxide synthase (eNOS). NO diffuses into the underlying smooth muscle cells and promotes relaxation and vasodilation. Other mediators include prostacyclin (PGI2), which also induces vasodilation, and endothelin-1, a potent vasoconstrictor. The balance between these opposing factors is critical for maintaining normal blood pressure and blood flow.
Barrier Function
The endothelium acts as a selective barrier, regulating the passage of substances between the bloodstream and surrounding tissues. This barrier function is essential for maintaining homeostasis and preventing excessive leakage of plasma proteins and fluids into the interstitial space. The integrity of the endothelial barrier is influenced by inflammatory cytokines and mechanical forces such as shear stress.
Inflammation and Immune Response
Endothelial cells play a critical role in the inflammatory response by expressing adhesion molecules such as selectins and integrins that facilitate the recruitment of leukocytes to sites of injury or infection. They produce pro-inflammatory cytokines and chemokines that attract immune cells and contribute to the local inflammatory milieu. The endothelium also participates in the resolution of inflammation by producing anti-inflammatory mediators.
Coagulation and Fibrinolysis
The endothelium is involved in the regulation of blood coagulation and fibrinolysis. Under normal conditions, the endothelium expresses anticoagulant factors, such as thrombomodulin and tissue factor pathway inhibitor, to prevent excessive clot formation. In response to injury or inflammation, the endothelium can switch to a pro-coagulant state, promoting platelet adhesion and clot formation. This dynamic regulation is essential for maintaining hemostasis and preventing thrombosis.
Angiogenesis
Endothelial cells are central to the process of angiogenesis, the formation of new blood vessels from pre-existing ones. This process is critical during development, wound healing, and in response to ischemia. Angiogenesis is regulated by several factors, including VEGF, fibroblast growth factor (FGF) and angiopoietins, which promote endothelial cell proliferation, migration and formation of new capillary structures.
Pathophysiology of the Vascular Endothelium
Dysfunction of the vascular endothelium is a key feature in the pathogenesis of various cardiovascular diseases, including atherosclerosis, hypertension, and diabetes.
Endothelial Dysfunction
Endothelial dysfunction is characterized by a reduced ability of the endothelium to regulate vascular tone, promote vasodilation, and maintain barrier integrity. This condition is often associated with an imbalance in the production of vasodilators and vasoconstrictors. Risk factors such as hyperlipidemia, hypertension, smoking, and diabetes can lead to endothelial damage and dysfunction. The consequences of endothelial dysfunction include increased vascular permeability, enhanced leukocyte adhesion, and a pro-inflammatory state, all of which contribute to the development of atherosclerosis.
Atherosclerosis
Atherosclerosis is a chronic inflammatory disease characterized by the accumulation of lipids, inflammatory cells and fibrous tissue in the arterial wall. The process begins with endothelial injury, which facilitates the entry of low-density lipoprotein (LDL) cholesterol into the intima and promotes the recruitment of monocytes, which differentiate into macrophages. These macrophages engulf oxidized LDL, become foam cells, and contribute to the formation of atherosclerotic plaques. The progression of atherosclerosis is characterized by further deterioration of endothelial function, leading to increased vascular stiffness and reduced blood flow.
Hypertension
Chronic hypertension can lead to structural changes in the endothelium and vascular remodeling. Elevated blood pressure induces mechanical stress on endothelial cells, leading to increased production of reactive oxygen species (ROS) and decreased NO bioavailability. This results in impaired vasodilation and exacerbates hypertension. Endothelial dysfunction in hypertension is also associated with inflammation and increased expression of adhesion molecules, promoting leukocyte recruitment and further vascular injury.
Diabetes
Diabetes mellitus is associated with significant endothelial dysfunction, characterized by impaired NO production, increased oxidative stress, and enhanced inflammation. Hyperglycemia leads to the formation of advanced glycation end products (AGEs), which can induce endothelial cell injury and promote atherosclerosis. Diabetic patients often exhibit a heightened risk of cardiovascular events due to these pathological changes in endothelial function.
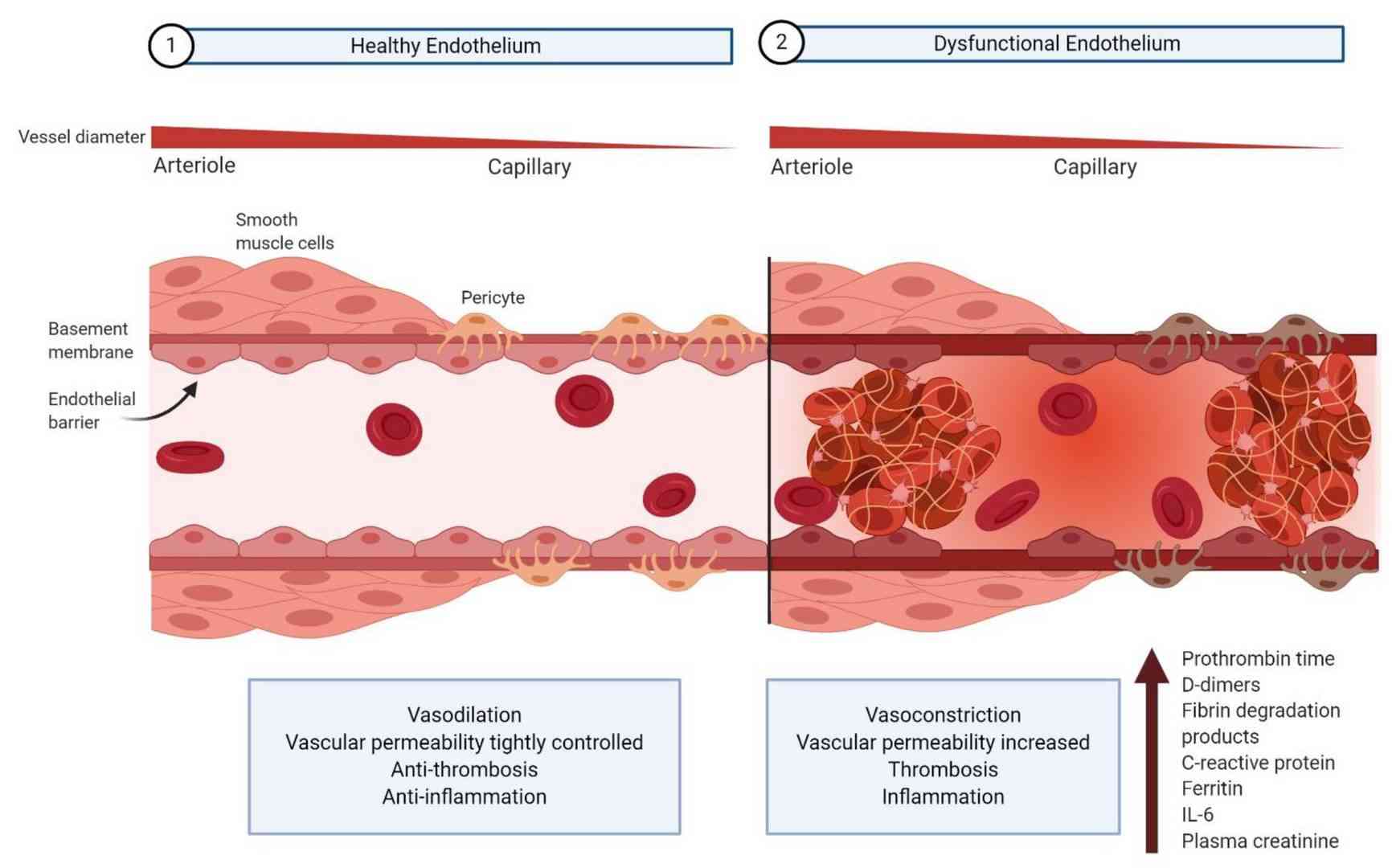
In summary, the vascular endothelium is a critical component of the cardiovascular system with multiple roles in regulating vascular tone, maintaining barrier integrity, and modulating inflammatory and coagulation processes. Understanding the structure and functions of the endothelium, as well as the mechanisms underlying endothelial dysfunction, is essential for the development of therapeutic strategies to prevent and treat cardiovascular disease. Continued research into the molecular and cellular pathways involved in endothelial biology will enhance our understanding of vascular health and disease and pave the way for novel interventions to restore endothelial function and improve cardiovascular outcomes.
Case Study
Case 1: Lanahan, A.; et al. PTP1b is a physiologic regulator of vascular endothelial growth factor signaling in endothelial cells. Circulation. 2014, 130(11), 902–909.
Vascular endothelial growth factor receptor 2 (VEGFR2) is a receptor tyrosine kinase responsible for regulating endothelial cell migration and proliferation, primarily in response to signals from various members of the vascular endothelial growth factor (VEGF) family. Recent studies have highlighted the importance of endothelial trafficking of VEGFR2 in controlling its activity and suggested the involvement of phosphotyrosine phosphatase 1b (PTP1b) in this process. This study was designed to elucidate the role of PTP1b in endothelial VEGFR2 signaling and its influence on angiogenesis and arteriogenesis. Mice with endothelial-specific deletion of PTP1b were generated to evaluate the effect of the knockout on VEGF signaling, angiogenesis and arteriogenesis both in vitro and in vivo. PTP1b knockout endothelial cells exhibited increased VEGF-dependent activation of extracellular signal-regulated kinase signaling as well as enhanced sprouting, migration, and proliferation compared to controls. These results indicate that PTP1b is a critical regulator of VEGFR2 signaling and significantly influences the extent of vascular network formation.
To evaluate the effect of PTP1b deletion on endothelial cell responses to various growth factors, primary endothelial cells (ECs) from PtpN1fl/fl mice were treated with either adenovirus expressing the Cre gene (Ad-Cre) or a control virus (Ad-CMV), then growth arrested and stimulated with IGF-1, VEGF-A165, or FGF2. As expected, deletion of PTP1b resulted in increased activation of the IGF1 receptor (IGF1Rβ) and ERK signaling (Fig. 4A and 4B). In support of the hypothesis that PTP1b downregulates VEGF signaling, VEGF-induced ERK activation was also increased in knockout ECs compared to wild type (Fig. 4C and 4D). However, no changes in ERK activation were observed after FGF2 stimulation, suggesting that PTP1b does not play a role in FGF signaling (Fig. 4E and 4F).
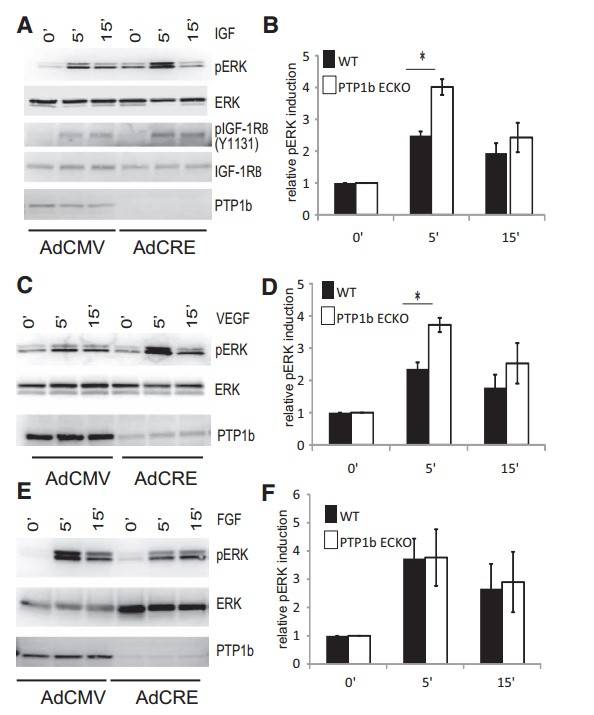
Case 2: Moss, M.; et al. The ADAM10 prodomain is a specific inhibitor of adam10 proteolytic activity and inhibits cellular shedding events. Journal of Biological Chemistry. 2007, 282(49), 35712–35721.
ADAM10 is a disintegrin metalloproteinase involved in the processing of amyloid precursor proteins and ErbB ligands, as well as the shedding of various type I and II membrane proteins. Similar to ADAM17 (TACE), ADAM10 is expressed as a zymogen whose activation requires removal of the prodomain. In this study, recombinant mouse ADAM10 prodomain purified from Escherichia coli was found to be a potent competitive inhibitor of the human ADAM10 catalytic/disintegrin domain.
Testing indicated that ADAM10 inhibition followed a competitive mechanism. A Lineweaver-Burk plot showed curved lines, prompting the use of a 1/v versus 1/[S2] plot, which fit well, showing varying slopes and consistent intercepts. This confirmed that the ADAM10 prodomain acts as a competitive inhibitor of the catalytic and disintegrin domains of the enzyme, with at least two binding sites for the fluorescent substrate. The velocity versus substrate data fit the competitive inhibitor model, reinforcing the inhibitory role of the prodomain.
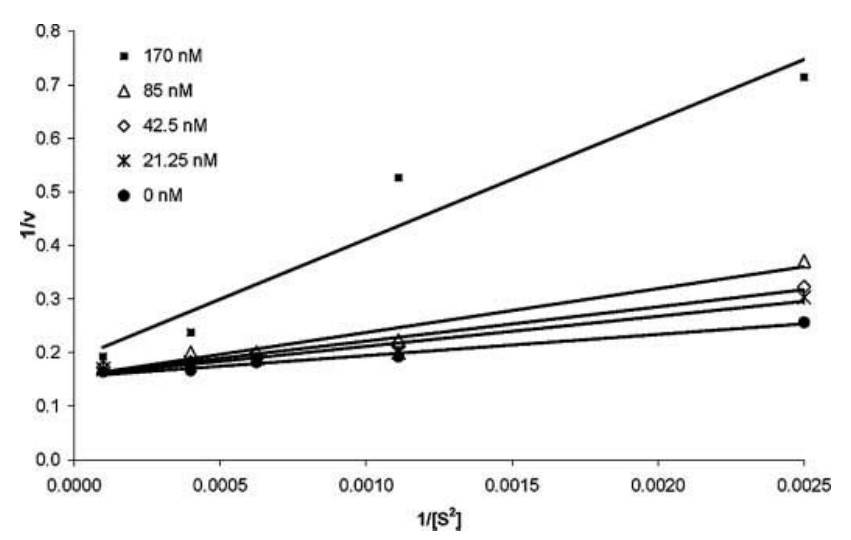
References
- Bernard, I., Limonta, D., Mahal, L., & Hobman, T. (2020). Endothelium infection and dysregulation by sars-cov-2: Evidence and caveats in covid-19. Viruses, 13(1), 29.
- Dela Paz, N. G., & D'Amore, P. A. (2009). Arterial versus venous endothelial cells. Cell and Tissue Research, 335(1), 5–16.
- Gu, X., & Cleaver, O. (2022). Angiodiversity—A tale retold by comparative transcriptomics. In The Vasculome (pp. 199–218). Elsevier.
- Lanahan, A. A., Lech, D., Dubrac, A., Zhang, J., Zhuang, Z. W., Eichmann, A., & Simons, M. (2014). Ptp1b is a physiologic regulator of vascular endothelial growth factor signaling in endothelial cells. Circulation, 130(11), 902–909.
- Moss, M. L., Bomar, M., Liu, Q., Sage, H., Dempsey, P., Lenhart, P. M., Gillispie, P. A., Stoeck, A., Wildeboer, D., Bartsch, J. W., Palmisano, R., & Zhou, P. (2007). The adam10 prodomain is a specific inhibitor of adam10 proteolytic activity and inhibits cellular shedding events. Journal of Biological Chemistry, 282(49), 35712–35721.
- Rajendran, P., Rengarajan, T., Thangavel, J., Nishigaki, Y., Sakthisekaran, D., Sethi, G., & Nishigaki, I. (2013). The vascular endothelium and human diseases. International Journal of Biological Sciences, 9(10), 1057–1069.