Immune System Development
Creative BioMart Immune System Development Product List
Immunology Background
Background
The immune system is a complex network of cells, tissues, and organs that work together to protect the body from pathogens, including bacteria, viruses, fungi, and parasites. Its development begins early in embryonic life and continues through childhood, becoming fully functional as the body matures. The development of the immune system is tightly regulated and involves numerous signaling pathways, transcription factors, and environmental cues.
Embryonic Origin of the Immune System
The immune system begins to form during early embryogenesis, with the first precursors of immune cells derived from hematopoietic stem cells (HSCs) in the yolk sac and later in the aorta-gonad-mesonephros (AGM) region. In the second trimester, the fetal liver becomes the primary site of hematopoiesis, producing the majority of early immune cells. Eventually, this role shifts to the bone marrow, which becomes the long-term source of all immune cell precursors.
The development of the immune system is divided into two main branches: the innate immune system and the adaptive immune system. The innate immune system is the body's first line of defense and consists of cells such as macrophages, neutrophils, and natural killer (NK) cells that provide immediate but unspecific protection. The adaptive immune system, on the other hand, includes highly specialized cells such as T and B lymphocytes that develop memory and provide long-term immunity.
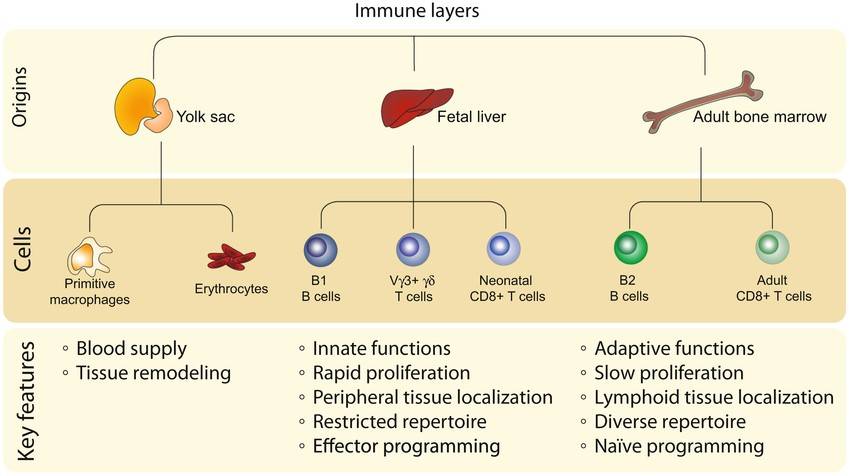
Development of Central Immune Organs
Two primary organs are essential for the development of immune cells: the bone marrow and the thymus. These organs are responsible for the maturation and differentiation of immune cells before they enter the bloodstream to perform their immune functions.
Bone Marrow
The bone marrow is the primary site of hematopoiesis in the adult and the origin of both innate and adaptive immune cells. It provides the environment necessary for HSCs to differentiate into the various blood cells, including red blood cells, platelets, and immune cells. Specifically, B cells mature in the bone marrow and undergo processes such as receptor gene rearrangement and negative selection to ensure self-tolerance and prevent autoimmunity.
Thymus
The thymus is critical for the maturation of T lymphocytes. Progenitor cells from the bone marrow migrate to the thymus where they undergo a highly regulated maturation process. This includes positive selection, which ensures that T cells can recognize major histocompatibility complex (MHC) molecules, and negative selection, which eliminates T cells that are highly reactive to self-antigens. The thymus reaches its maximum size during adolescence and then undergoes involution, decreasing in size and function with age. However, the population of mature T cells remains stable throughout adulthood due to the longevity of T cells and peripheral proliferation.
Development of Peripheral Immune Organs
Peripheral immune organs, such as the spleen, lymph nodes, and mucosa-associated lymphoid tissue (MALT), serve as sites for immune cell activation and coordination during immune responses.
Lymph Nodes
Lymph nodes are distributed throughout the body and act as filtration units where antigens are presented to immune cells. During fetal development, lymph nodes are seeded with lymphoid precursors that later differentiate into functional lymphocytes. After birth, lymph nodes play a critical role in adaptive immunity by hosting interactions between antigen presenting cells (APCs) and lymphocytes, facilitating the activation and differentiation of T and B cells.
Spleen
The spleen develops from mesodermal cells during embryogenesis and is important for filtering blood, removing old or damaged red blood cells, and responding to blood-borne pathogens. It is also an important site for B-cell activation and antibody production.
Mucosa-Associated Lymphoid Tissue (MALT)
MALT includes the tonsils, Peyer's patches in the intestines, and other lymphoid tissues associated with mucosal surfaces. These tissues form early in life and provide the first line of immune defense at mucosal barriers, which are often the entry points for pathogens. They contain specialized cells that sample antigens from the environment and present them to lymphocytes, thereby initiating immune responses.
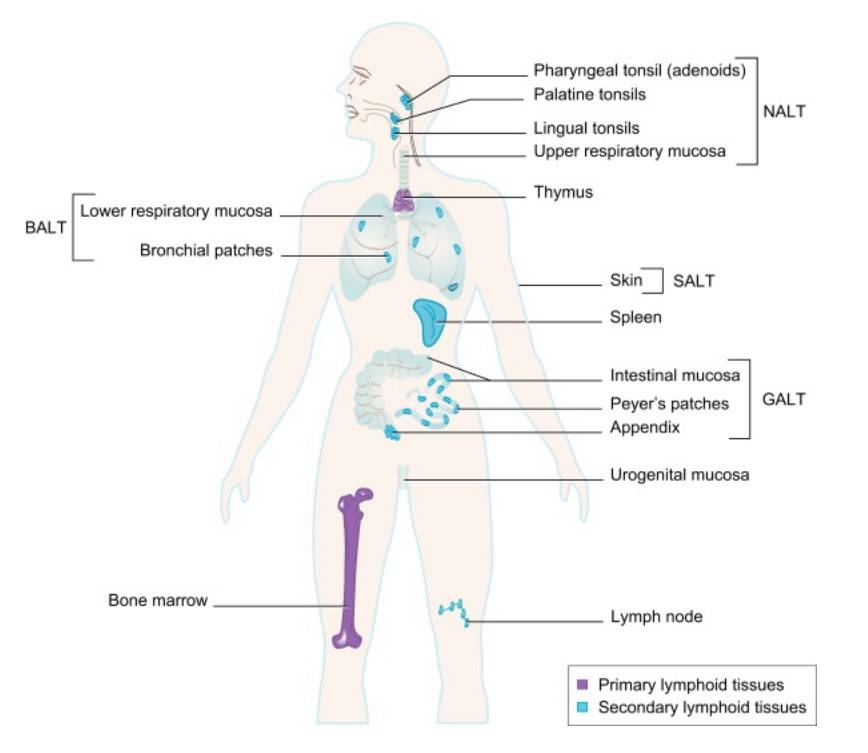
Differentiation and Maturation of Immune Cell Lineages
The immune system is composed of different cell types, each with specific functions. The differentiation and maturation of these cells is critical for the development of both innate and adaptive immunity.
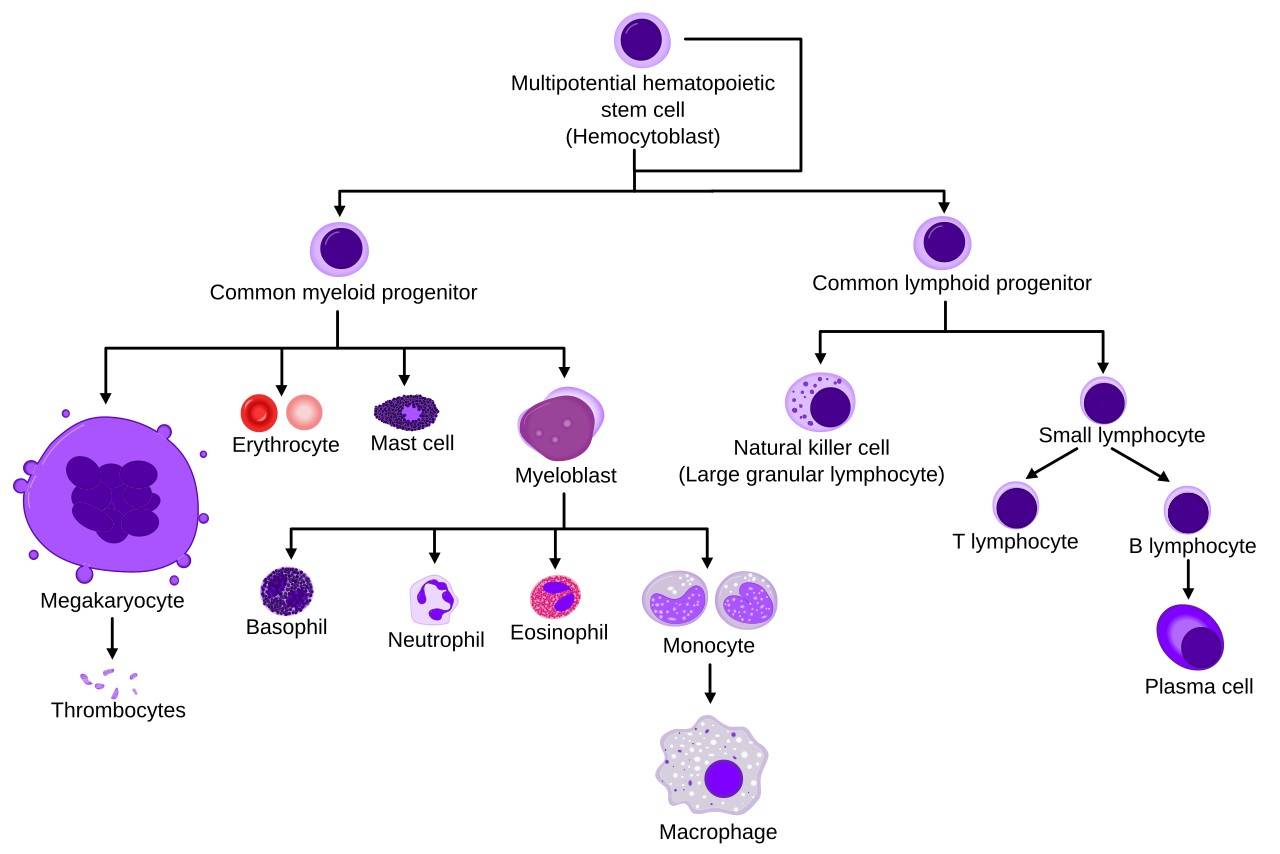
Innate Immune Cells
Innate immune cells, including macrophages, neutrophils, dendritic cells, and NK cells, develop from myeloid precursors in the bone marrow. These cells are equipped with pattern recognition receptors (PRRs) that allow them to recognize conserved pathogen-associated molecular patterns (PAMPs) on microorganisms. The development of these cells is tightly regulated by cytokines and transcription factors, such as interferon regulatory factors (IRFs) for NK cells and colony stimulating factors (CSFs) for granulocytes and macrophages.
Adaptive Immune Cells
B and T lymphocytes, the key players in adaptive immunity, develop from lymphoid progenitors. B cells mature in the bone marrow, where they rearrange their immunoglobulin genes to generate diverse antibody specificities. After encountering an antigen, B cells can differentiate into plasma cells, which produce antibodies, or memory B cells, which provide long-term immunity.
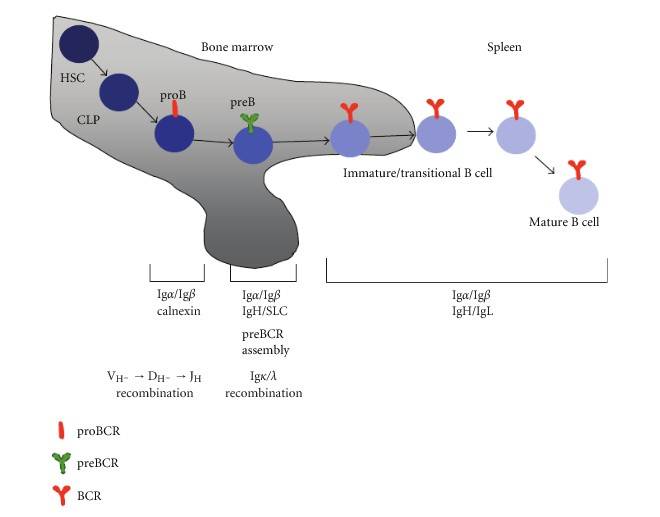
T cells mature in the thymus, where they undergo a rigorous selection process to ensure that only functional and self-tolerant cells are released into the circulation. Naive T cells can differentiate into various effector subsets, including helper T cells (Th), cytotoxic T cells (CTLs), and regulatory T cells (Tregs), each with distinct roles in immune defense and tolerance.
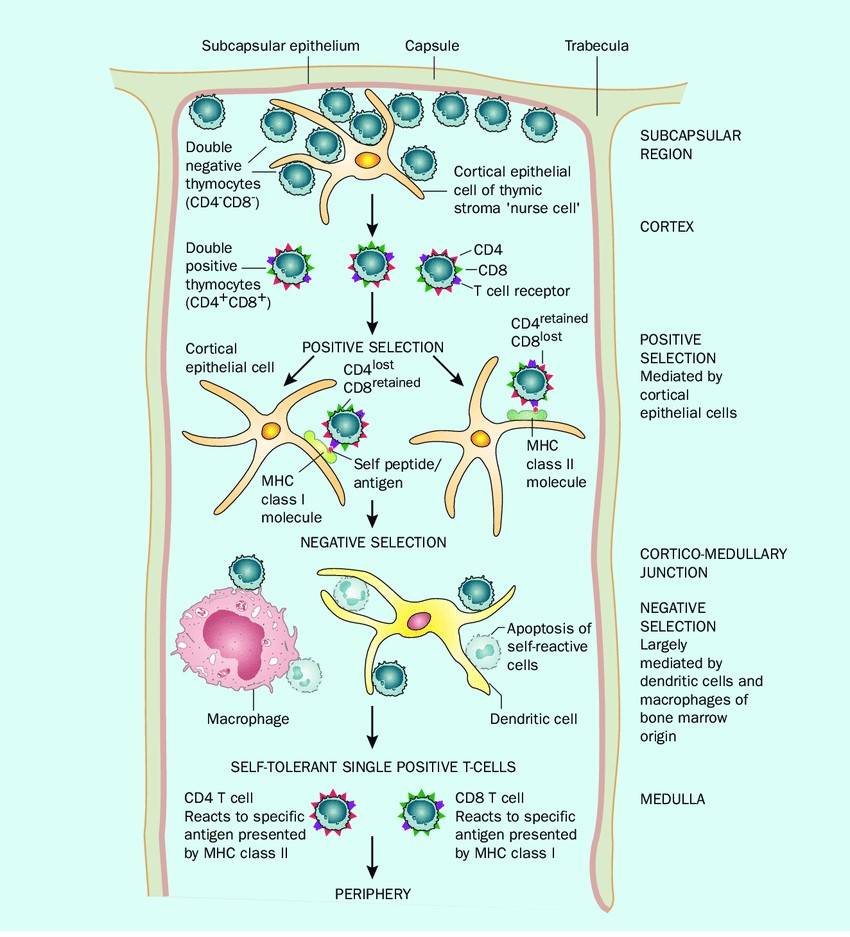
Regulation of Immune System Development
The development of the immune system is influenced by both intrinsic genetic programs and extrinsic environmental factors. Several transcription factors, such as GATA-3, PU.1 and NF-κB, regulate the lineage commitment and differentiation of immune cells. In addition, cytokines and growth factors, such as interleukin-7 (IL-7) for lymphocytes and granulocyte-macrophage colony-stimulating factor (GM-CSF) for myeloid cells, are essential for immune cell development.
Environmental factors, including microbial exposure and diet, also play an important role in shaping the immune system. For example, exposure to pathogens during infancy helps train the immune system to discriminate between harmful and harmless antigens. This is critical for the development of immune tolerance, which prevents overreaction to non-threatening stimuli such as food or self-antigens.
Immune Cell Markers
Immune cell markers, also known as surface markers or cluster of differentiation (CD) molecules, are proteins or glycoproteins expressed on the surface of immune cells that are used to identify and differentiate between various cell types. These markers play critical roles in cell signaling, adhesion, and immune responses, and they are widely utilized in immunology to track the development, differentiation, and activation states of immune cells.
Hematopoietic Progenitor Cell Markers
Hematopoietic stem cells are the progenitors of all immune cells. Key markers for identifying HSCs include:
- CD34: This is the primary marker of HSCs, present in both human and mouse HSCs.
- CD117 (c-Kit): Another important marker for identifying multipotent progenitor cells.
- Sca-1: A stem cell antigen commonly used in conjunction with CD34 to identify mouse HSCs.
Myeloid Lineage Markers
Myeloid cells include a range of innate immune cells such as macrophages, neutrophils, dendritic cells, and monocytes. Important markers for myeloid lineage cells are:
- CD11b: Expressed on monocytes, macrophages, and granulocytes, it is involved in cell adhesion and migration.
- CD14: Primarily expressed on monocytes and macrophages, it acts as a co-receptor for detecting bacterial lipopolysaccharide (LPS).
- CD16 (FcγRIII, CD16a and CD16b): Found on neutrophils, NK cells, and some macrophages, it mediates antibody-dependent cellular cytotoxicity.
- CD33: A sialic acid-binding immunoglobulin-like lectin (Siglec) expressed on myeloid progenitors and mature myeloid cells, including monocytes and macrophages.
Lymphoid Lineage Markers
Lymphoid cells include T cells, B cells, and NK cells, which are key players in adaptive immunity. These cells express specific surface markers that reflect their lineage and functional status:
T Cell Markers
T lymphocytes (T cells) are responsible for cell-mediated immunity. They can be classified into various subsets based on their function, with unique markers indicating each subtype:
- CD3: A core marker of all T cells, part of the T-cell receptor (TCR) complex.
- CD4: Expressed on helper T cells (Th cells), which assist in activating other immune cells.
- CD8: Found on cytotoxic T cells (Tc cells), responsible for directly killing infected or cancerous cells.
- CD25: The alpha chain of the IL-2 receptor, expressed on activated T cells and regulatory T cells (Tregs).
- FOXP3: A transcription factor critical for the function and development of regulatory T cells.
B Cell Markers
B lymphocytes (B cells) are responsible for humoral immunity, producing antibodies after activation. Key markers for B cells include:
- CD19: A pan-B cell marker involved in B cell activation and development.
- CD20: Expressed on mature B cells and often targeted in therapeutic approaches for B cell malignancies.
- CD21: The receptor for complement protein C3d, expressed on mature B cells and involved in immune complex recognition.
- CD38: Found on activated and plasma B cells, marking the transition from B cells to antibody-secreting cells.
Natural Killer Cells (NK Cells) Markers
NK cells are part of the innate immune system and are involved in the direct killing of infected or cancerous cells. Important NK cell markers include:
- CD56: A defining marker for human NK cells.
- CD16 (FcγRIII): Also present on NK cells, it plays a key role in antibody-dependent cellular cytotoxicity.
- NKG2D: A receptor on NK cells that recognizes stress-induced ligands on target cells.
Dendritic Cell Subsets and Lineage-specific Markers
Dendritic cells are professional antigen-presenting cells (APCs) that play a central role in initiating adaptive immune responses. Their key markers include:
- CD11c: A characteristic marker of myeloid dendritic cells, involved in antigen presentation.
- CD80 and CD86: Co-stimulatory molecules that are essential for T cell activation.
- HLA-DR: A major histocompatibility complex (MHC) class II molecule involved in presenting antigens to CD4+ T cells.
Macrophage Markers
Macrophages are highly phagocytic cells that contribute to both innate and adaptive immune responses. Their markers include:
- CD68: A pan-macrophage marker, used to identify macrophages in tissues.
- CD163: A scavenger receptor for hemoglobin-haptoglobin complexes, associated with anti-inflammatory functions.
- F4/80: A specific marker for mouse tissue-resident macrophages.
Monocyte Markers
Monocytes circulate in the blood and can differentiate into macrophages or dendritic cells upon migration to tissues. Important markers for monocytes include:
- CD14: A co-receptor for the detection of LPS and marker for classical monocytes.
- CD16: Used to identify non-classical monocytes with different functional roles compared to CD14+ monocytes.
Clinical Implications of Immune System Development
Defects in the development of the immune system can lead to immunodeficiency, autoimmunity or hypersensitivity disorders. Primary immunodeficiency diseases (PIDs), such as severe combined immunodeficiency (SCID) and DiGeorge syndrome, result from genetic mutations that affect the development or function of immune cells. These disorders leave individuals vulnerable to infections and other complications.
On the other hand, dysregulation of immune system development can lead to autoimmune diseases in which the immune system mistakenly attacks the body's own tissues. For example, errors in negative selection during T cell development can lead to the survival of self-reactive T cells, contributing to diseases such as type 1 diabetes and systemic lupus erythematosus.
Hypersensitivity reactions, such as allergies, can also result from an overactive immune response to innocuous environmental antigens. The hygiene hypothesis suggests that reduced microbial exposure in early life may contribute to the increased prevalence of allergic diseases in developed countries by impairing immune tolerance mechanisms.
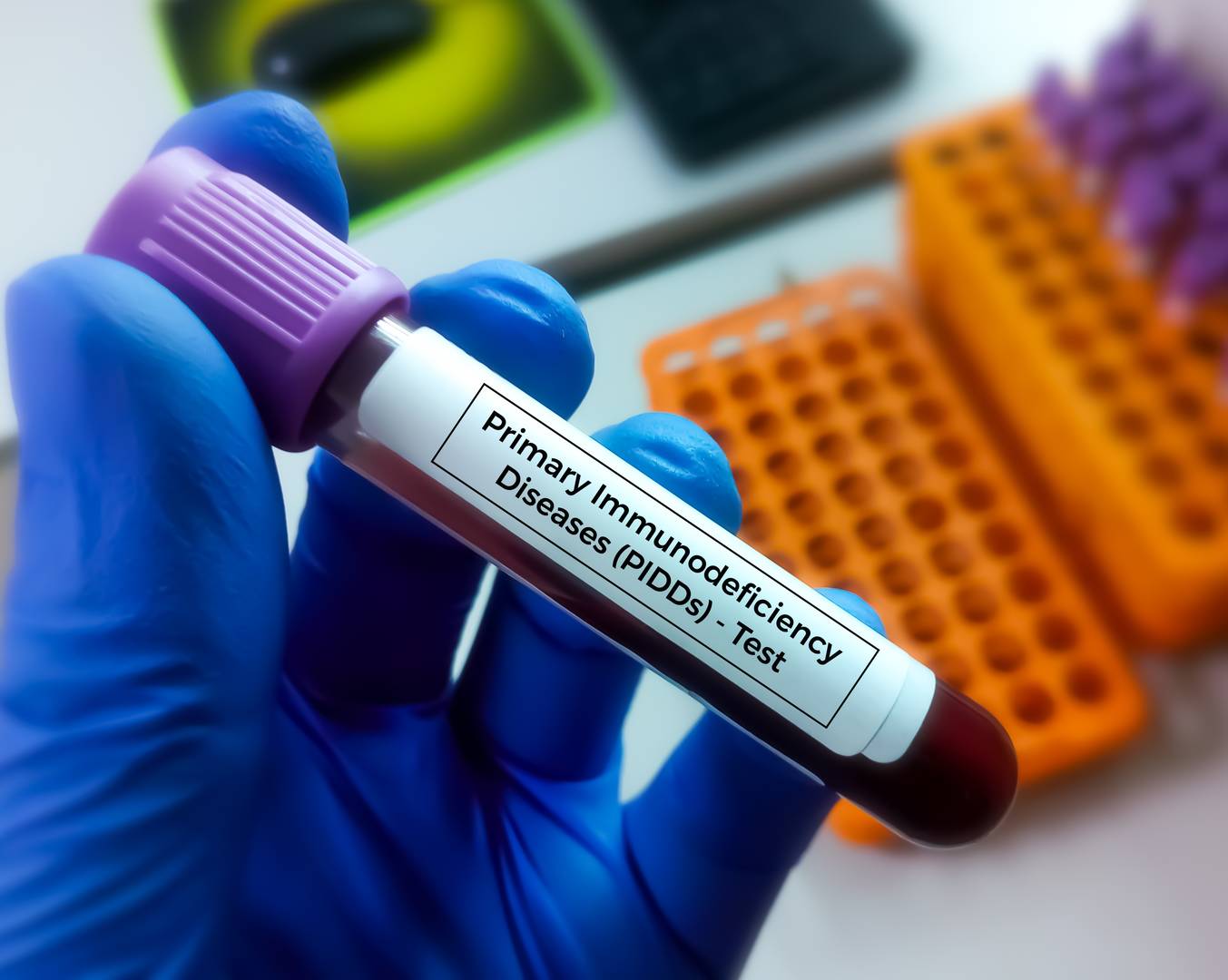
In summary, the development of the immune system is a complex and highly regulated process involving the coordinated differentiation of multiple cell lineages, the establishment of central and peripheral immune organs, and the interaction of genetic and environmental factors. From the early stages of hematopoiesis to the maturation of immune cells and the formation of a functional immune network, each step is critical for maintaining the body's defense against pathogens while preventing self-reactivity. Understanding the intricacies of immune system development not only provides insight into normal immune function, but also has important implications for the treatment of immunological disorders such as immunodeficiency, autoimmunity and allergy.
Case Study
Case 1: Thorarinsdottir, K.; et al. CD21-/low B cells: A snapshot of a unique b cell subset in health and disease. Scandinavian Journal of Immunology. 2015, 82(3), 254–261.
B cells are immune cells that protect against pathogens by differentiating into plasma cells to produce antibodies. In autoimmune diseases, they can produce autoantibodies that attack and damage self-tissues. Although historically considered secondary to T cells in autoimmune pathology, successful treatments targeting B cells (such as rituximab and belimumab) have highlighted their broader role. Recent research has identified several B cell subsets, including regulatory and memory B cells, with unique functions in conditions such as autoimmune diseases, chronic viral infections, and immunodeficiencies. One subset, CD21-/low B cells, expands in response to chronic immune stimulation and this review will focus on their characteristics.
Tab. 1: Definition and characterization of CD21-/low cell subsets in different conditions.
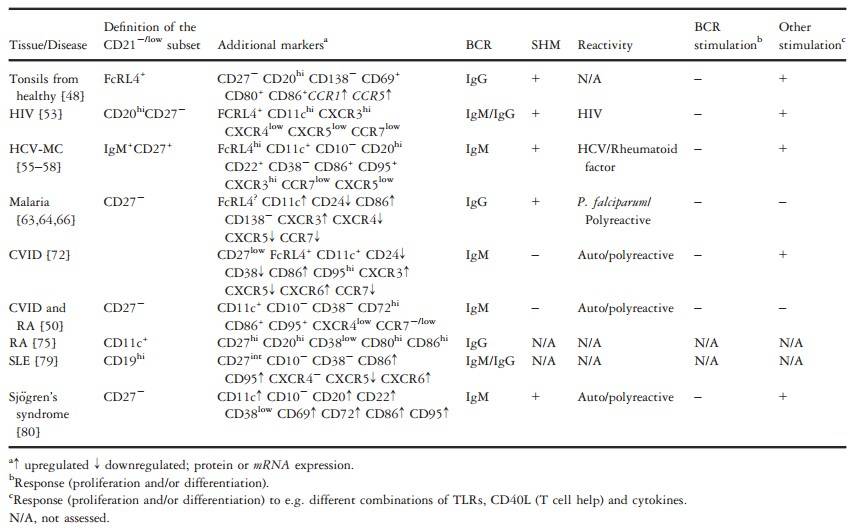
Case 2: Hilpert, J.; et al. Comprehensive analysis of NKG2D ligand expression and release in leukemia: Implications for NKG2D-mediated NK cell responses. The Journal of Immunology. 2012, 189(3), 1360–1371.
NKG2D ligands on cancer cells make them vulnerable to NK cell-mediated destruction, but cancer cells can evade this through shedding of these ligands, reducing surface expression and NK reactivity. In leukemia patients, 75% of leukemia cells expressed at least one NKG2D ligand on the surface, with all patients showing high levels of soluble NKG2D ligands (sNKG2DL) in their sera, which downregulate NKG2D on NK cells and impair antileukemia response. This study highlights how NKG2D/NKG2DL interactions influence NK cell function and immune evasion in leukemia.
To analyze the influence of NKG2DL expression on NK antileukemia reactivity, they used primary AML, ALL, and CLL cells as targets. Blocking the interaction between NKG2D and its ligands significantly reduced NK cell-mediated lysis of leukemia cells. When multiple NKG2DL were present, blocking one reduced NK lysis, with additive effects seen when multiple ligands were blocked. In addition to cytotoxicity, NKG2D ligands also promoted cytokine production (IFN-g) by NK cells, further emphasizing their importance in NK cell responses against leukemia.
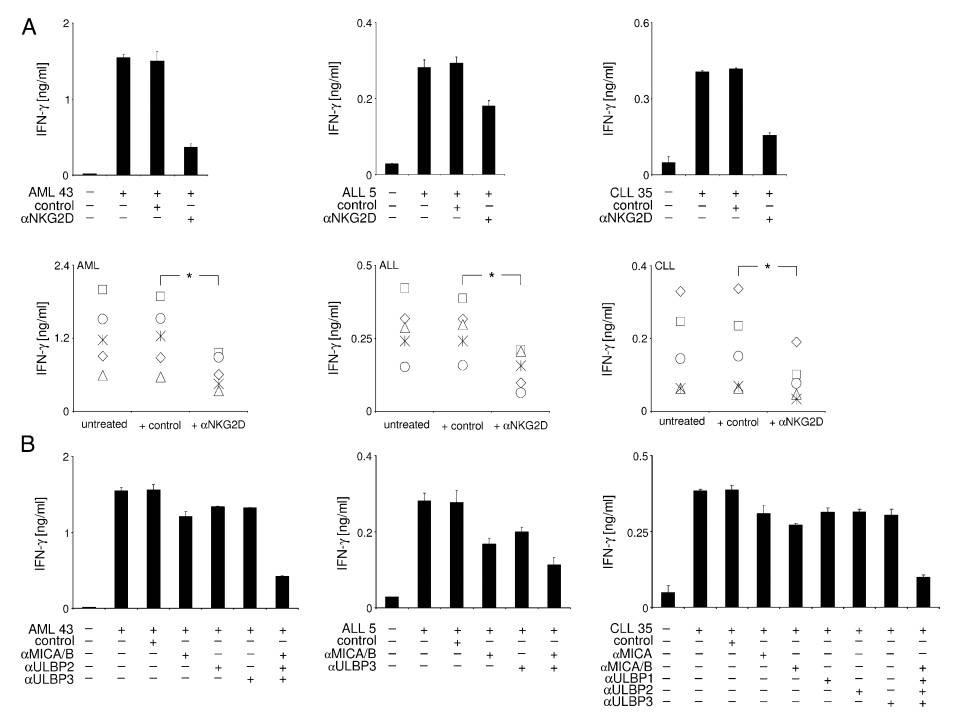
References
- Primer to the immune response, 2. Edition, 2014. Elsevier.
- Hilpert, J., Grosse-Hovest, L., Grünebach, F., Buechele, C., Nuebling, T., Raum, T., Steinle, A., & Salih, H. R. (2012). Comprehensive analysis of nkg2d ligand expression and release in leukemia: Implications for nkg2d-mediated NK cell responses. The Journal of Immunology, 189(3), 1360–1371.
- Luria-Pérez, R., Sánchez-Vargas, L. A., Muñoz-López, P., & Mellado-Sánchez, G. (2022). Mucosal vaccination: A promising alternative against flaviviruses. Frontiers in Cellular and Infection Microbiology, 12, 887729.
- Pérez-Vera, P., Reyes-León, A., & Fuentes-Pananá, E. M. (2011). Signaling proteins and transcription factors in normal and malignant early b cell development. Bone Marrow Research, 2011, 1–10.
- Storey, M., & Jordan, S. (2008). An overview of the immune system. Nursing Standard, 23(15), 47–56.
- Tabilas, C., Smith, N. L., & Rudd, B. D. (2023). Shaping immunity for life: Layered development of CD8+ T cells. Immunological Reviews, 315(1), 108–125.
- Thorarinsdottir, K., Camponeschi, A., Gjertsson, I., & Mårtensson, I. L. (2015). cd 21−/low b cells: A snapshot of a unique b cell subset in health and disease. Scandinavian Journal of Immunology, 82(3), 254–261.