Peptidylarginine Deiminases
Related Symbol Search List
Immunology Background
Background
Peptidylarginine deiminases (PADs) are a family of enzymes that catalyze the conversion of peptidylarginine residues to peptidylcitrulline through a process called citrullination or deimination.
The number of PAD isotypes increases during evolution from one in fishes and amphibians, three in reptiles and chicken, to five in mammals, including human (called PAD1, 2, 3, 4, and 6, encoded by the corresponding PADI1-4 and 6 genes). PADs are 663–665 amino acids long proteins with a molecular mass of ~74 kDa, except PAD6 that contains 694 amino acids.
The expression and activity of PADs are regulated at multiple levels, including transcriptional, translational, and post-translational levels. The first and so far only biological regulator of PAD, namely the tyrosine-protein phosphatase non-receptor type 22 (PTPN22), was recently characterized as a non-enzymatic inhibitor of PAD4.
Deimination or citrullination
PADs replace the primary ketimine group of arginine (=NH) by a ketone one (=O) and yield ammonia as a side-product (Figure 1a). Since arginine is positively charged at a neutral pH, whereas, citrulline is not, deimination induces a decreased net charge of targeted proteins; this can change their hydrophobicity, folding, and intra- or inter-molecular ionic interactions, leading to changes in their function and their fate.
Deimination is involved in a growing number of physiological processes (innate and adaptive immunity, control of gene expression, embryonic development, etc.) and has been associated with several human diseases (cancer, rheumatoid arthritis, neurodegenerative diseases, etc.).
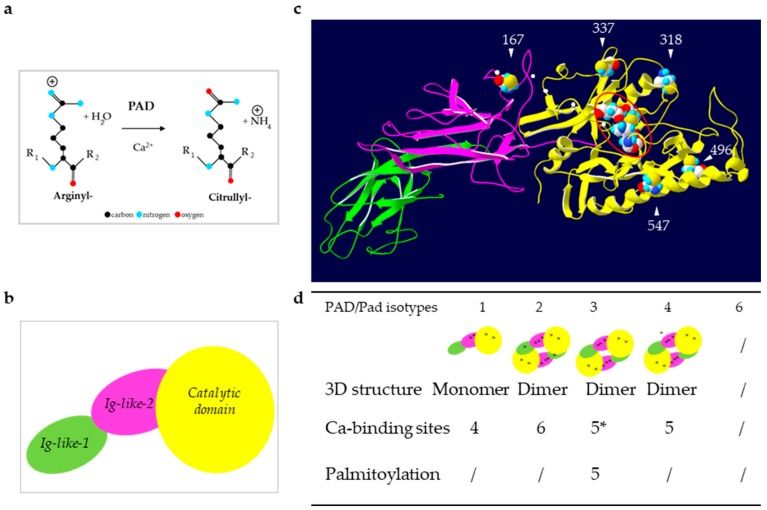
(a) Schematic representation of the reaction catalyzed by PADs: Deimination or citrullination. (b) Schematic representation of the sub-domains of PADs. (c) Illustration of an in silico three-dimensional (3D) model of the active PAD3. (d) Summary of the structural data for each human (PAD) and mouse (mPad) isotypes.
Role and Function of PADs
PAD enzymes play important roles in various physiological and pathological processes. Here's an overview of their role and functions
1. Protein Citrullination
The primary function of PAD enzymes is to catalyze the citrullination of proteins. This modification alters the charge and structure of target proteins, leading to functional changes. Citrullination can affect protein-protein interactions, enzymatic activity, protein stability, and subcellular localization. It plays a role in diverse cellular processes such as gene regulation, signal transduction, cytoskeletal organization, and immune responses.
2. Gene Regulation
PAD enzymes can influence gene expression by citrullinating histones, which are proteins that package DNA into a compact structure called chromatin. Citrullination of histones can result in changes to chromatin structure and accessibility, affecting the binding of transcription factors and the regulation of gene transcription. PAD4, in particular, has been implicated in the regulation of gene expression in immune cells and the development of autoimmune diseases.
3. Immune Responses
PAD enzymes play a critical role in immune responses and inflammation. They are involved in the citrullination of proteins in immune cells, affecting their function and interactions. PAD4-mediated citrullination is associated with the formation of neutrophil extracellular traps (NETs), which are web-like structures composed of citrullinated proteins and DNA released by neutrophils to trap and kill pathogens. PADs also contribute to the activation of immune cells and the generation of immune responses.
4. Tissue Homeostasis and Development
PAD enzymes are involved in tissue homeostasis and development. They play roles in processes such as epidermal differentiation, hair follicle development, and spermatogenesis. For example, PAD1 and PAD3 are primarily expressed in the epidermis and contribute to the formation and maintenance of the skin's protective barrier. In the testes, PAD6 is involved in spermatogenesis and the post-translational modification of sperm proteins.
5. Disease Associations
Dysregulation of PAD enzymes has been implicated in various diseases. PAD activity and citrullination have been linked to autoimmune diseases, including rheumatoid arthritis and systemic lupus erythematosus, where increased PAD activity and enhanced citrullination contribute to the pathogenesis of these conditions. PADs are also associated with neurodegenerative diseases, cancer, and cardiovascular diseases, highlighting their involvement in disease processes.
Understanding the role and function of PAD enzymes is crucial for unraveling their contributions to normal physiology and disease pathogenesis. Further research into the specific substrates, regulatory mechanisms, and cellular functions of PADs will provide valuable insights into their therapeutic potential and the development of targeted interventions for various diseases.
PADs and Histone Modifying Enzymes
PADs and histone modifying enzymes are two classes of enzymes that play important roles in post-translational modifications of proteins, specifically histones. While both PADs and histone modifying enzymes can impact gene regulation and chromatin structure, they differ in their specific modifications and functions. Here's an overview of the relationship between PADs and histone modifying enzymes:
Relationship | Details |
---|---|
PADs and Histone Citrullination | PAD enzymes, particularly PAD4, are responsible for the citrullination of histones, which involves the conversion of arginine residues to citrulline. This post-translational modification, known as histone citrullination, can alter chromatin structure and gene expression. Citrullination of histones by PADs can impact various aspects of gene regulation, including transcriptional activation or repression, recruitment of transcription factors, and modulation of chromatin accessibility. |
Histone Modifying Enzymes | Histone modifying enzymes, on the other hand, encompass a diverse group of enzymes that catalyze various chemical modifications on histone proteins, including acetylation, methylation, phosphorylation, ubiquitination, and more. These modifications are known as histone marks and play crucial roles in regulating chromatin structure, gene expression, and other chromatin-associated processes. Histone modifying enzymes include histone acetyltransferases (HATs), histone deacetylases (HDACs), histone methyltransferases (HMTs), histone demethylases (HDMs), and many others. |
Cross-Talk between PADs and Histone Modifying Enzymes | There is growing evidence of cross-talk and interplay between PADs and histone modifying enzymes. For example, PAD-mediated histone citrullination can impact the activities of histone-modifying enzymes and their ability to modify histones at specific sites. Citrullination of arginine residues in histones can influence the recognition and binding of histone modifying enzymes, thereby affecting their enzymatic activity and the establishment or removal of other histone marks. |
Combined Impact on Gene Regulation | The interplay between PADs and histone modifying enzymes can have significant implications for gene regulation. The combined effects of histone citrullination and other histone modifications can shape chromatin structure, modulate the recruitment of transcriptional regulators, and influence gene expression patterns. The specific interplay between PADs and histone modifying enzymes likely varies depending on the cellular context, the target genes involved, and the specific modifications being considered. |
Understanding the complex interactions between PADs and histone modifying enzymes is an active area of research. Further investigations into the cross-talk between these enzymes will provide insights into the regulation of chromatin dynamics, gene expression, and their implications in various physiological and pathological processes.
Members and Functions of PADs
There are five known members of the PAD family in humans: PAD1, PAD2, PAD3, PAD4, and PAD6. Here's an overview of their functions:
Members | Details |
---|---|
PAD1 | PAD1 is primarily expressed in the epidermis, specifically in the granular layer of the skin. It plays a crucial role in the terminal differentiation of keratinocytes, contributing to the formation of the skin's protective barrier. PAD1 citrullinates proteins involved in the cornified cell envelope, which helps stabilize the structure of the skin. |
PAD2 | PAD2 exhibits a broad expression pattern and is found in various tissues and cell types. It is particularly abundant in the central nervous system (CNS). PAD2 is involved in diverse processes, including neuronal differentiation, myelination, and regulation of gene expression. It citrullinates histones and other proteins, influencing chromatin structure and transcriptional activity. PAD2 has also been implicated in cancer progression, inflammation, and autoimmune diseases. |
PAD3 | Similar to PAD1, PAD3 is predominantly expressed in the epidermis, specifically in the granular layer. It plays a role in the cross-linking of structural proteins in the cornified cell envelope, contributing to the barrier function of the skin. PAD3 citrullinates certain proteins involved in the cornified envelope, affecting their stability and function. |
PAD4 | PAD4 is expressed in various tissues, including immune cells, skeletal muscle, and reproductive organs. Its main function is the citrullination of histones, leading to chromatin decondensation and regulation of gene expression. PAD4 is involved in the formation of neutrophil extracellular traps (NETs), which are web-like structures composed of DNA and citrullinated proteins released by neutrophils during immune responses. Dysregulation of PAD4 has been implicated in autoimmune diseases, such as rheumatoid arthritis and systemic lupus erythematosus. |
PAD6 | PAD6 is primarily expressed in the testes, particularly in developing spermatocytes. It plays a role in spermatogenesis and is involved in the post-translational modification of sperm proteins through citrullination. The exact functions and targets of PAD6 in the context of spermatogenesis are still being investigated. |
The expression patterns of PAD enzymes can vary depending on the tissue type, developmental stage, and physiological conditions. Their activities are tightly regulated to maintain proper cellular functions and prevent aberrant citrullination. They are involved in the regulation of gene expression, epidermal differentiation, immune responses, and reproductive functions. Understanding the specific functions of each PAD member contributes to our knowledge of their roles in normal physiology and their potential implications in diseases.
The Role and Mechanism of PADs in the Occurrence and Development of the Disease
PADs play a significant role in the occurrence and development of various diseases. Dysregulation of PAD activity and aberrant protein citrullination have been associated with several pathological conditions. Here are some examples illustrating the role and mechanisms of PADs in specific diseases:
Rheumatoid Arthritis (RA)
RA is an autoimmune disease characterized by chronic inflammation of the joints. PAD enzymes, particularly PAD4, have been implicated in the pathogenesis of RA. In this disease, increased PAD activity leads to the citrullination of proteins in joint tissues. Citrullinated proteins, such as fibrinogen and collagen, become targets of the immune system, leading to the production of autoantibodies called anti-citrullinated protein antibodies (ACPAs). ACPAs contribute to the chronic inflammation, immune cell recruitment, and destruction of joint tissues observed in RA.
Systemic Lupus Erythematosus (SLE)
SLE is a systemic autoimmune disease characterized by immune dysregulation and the production of autoantibodies against self-antigens. PADs, especially PAD4, are involved in the generation of autoantigens in SLE. PAD4-mediated citrullination of histones in immune cells, such as neutrophils, leads to the formation of neutrophil extracellular traps (NETs). NETs contain citrullinated histones and DNA, which can trigger an immune response and contribute to the development of autoimmunity in SLE.
Cancer
PADs have been implicated in various types of cancer, including breast, lung, and colon cancer. Aberrant PAD expression and citrullination have been observed in cancer cells and tumor tissues. In cancer, PADs can influence tumor cell behavior through multiple mechanisms. For example, PAD-mediated citrullination of cytoskeletal proteins, such as vimentin, can promote tumor cell migration and invasion. Citrullination of histones can affect chromatin structure and gene expression, potentially impacting cell growth and survival pathways.
Neurodegenerative Diseases
PADs have been associated with neurodegenerative diseases such as Alzheimer's disease (AD) and multiple sclerosis (MS). In AD, increased PAD activity and citrullination have been observed in the brains of patients. PAD-mediated citrullination can promote the aggregation and toxicity of proteins involved in AD, including beta-amyloid and tau. In MS, citrullination of myelin proteins may contribute to immune responses against myelin and contribute to the demyelination observed in the disease.
Cardiovascular Diseases
PADs have been implicated in cardiovascular diseases, including atherosclerosis and vascular calcification. In atherosclerosis, PAD-mediated citrullination of proteins in the vessel wall can contribute to chronic inflammation and the formation of atherosclerotic plaques. Citrullination of extracellular matrix proteins may also promote vascular calcification, a process associated with arterial stiffness and cardiovascular complications.
These examples highlight the diverse roles of PADs in different diseases. The mechanisms by which PADs contribute to disease pathogenesis involve protein citrullination, immune dysregulation, inflammation, and alterations in cellular processes. Understanding the specific contributions of PADs in different diseases can potentially lead to the development of targeted therapies for these conditions.
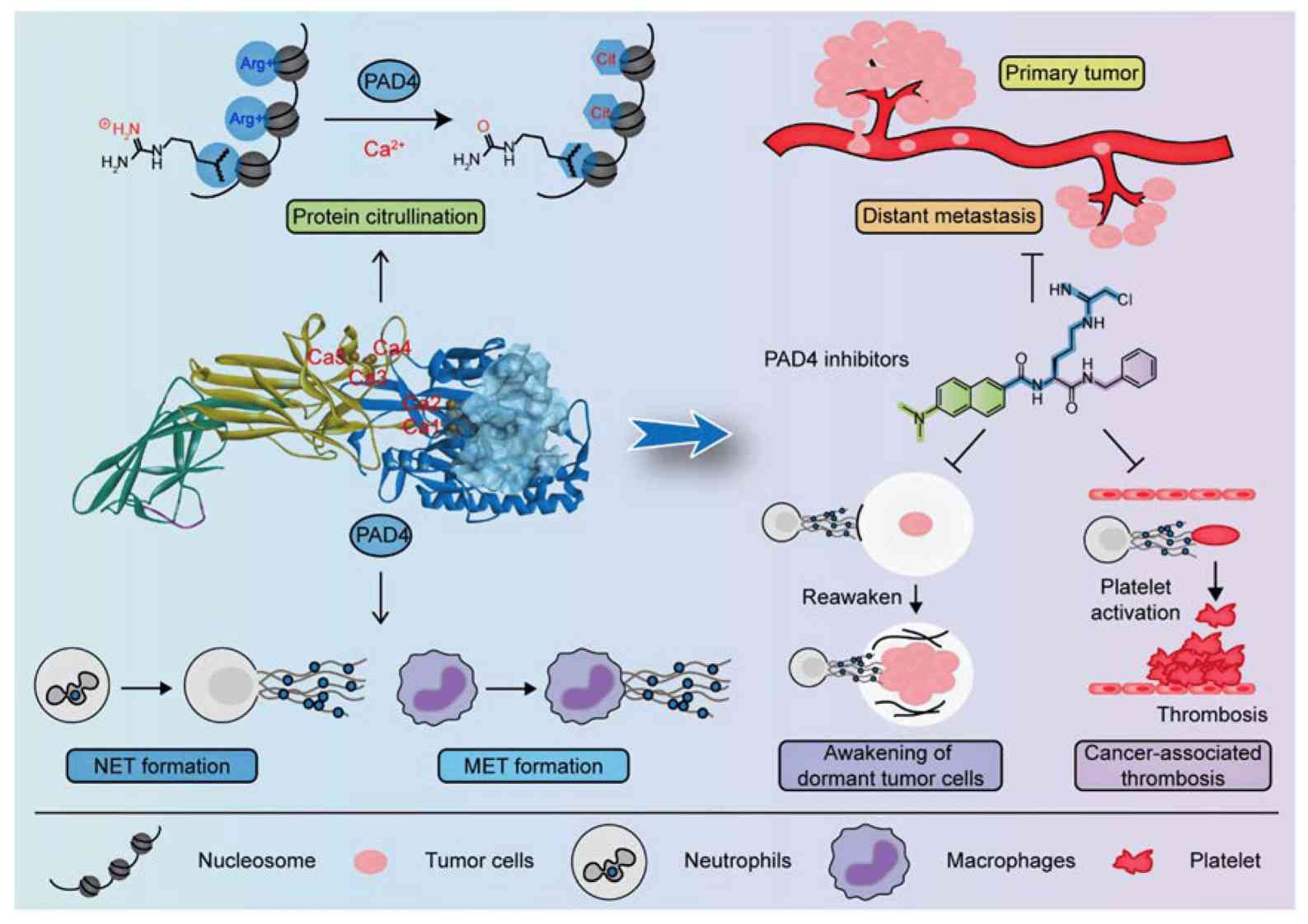
Case Study
Case 1: Pitter MR, Kryczek I, Zhang H, et al. PAD4 controls tumor immunity via restraining the MHC class II machinery in macrophages. Cell Rep. 2024;43(3):113942.
Tumor-associated macrophages (TAMs) play a crucial role in shaping tumor immunity and therapeutic response. However, the impact of post-translational modifications (PTMs) on TAM phenotype and function remains poorly understood. This study focuses on the role of peptidylarginine deiminase 4 (PAD4), an enzyme involved in PTMs, in TAMs and its correlation with immune checkpoint blockade response.
The researchers found that PAD4 exhibits the highest expression among common PTM enzymes in TAMs and negatively correlates with clinical response to immune checkpoint blockade therapy. Inhibiting PAD4 genetically or pharmacologically in macrophages prevented tumor progression in mouse models, accompanied by increased expression of macrophage major histocompatibility complex (MHC) class II and enhanced T cell effector function. Mechanistically, PAD4 was found to citrullinate STAT1 at arginine 121. This citrullination promoted the interaction between STAT1 and protein inhibitor of activated STAT1 (PIAS1). In the absence of PAD4, this interaction was abolished, resulting in the loss of the inhibitory role of PIAS1 in the expression of MHC class II machinery in macrophages. As a result, T cell activation was enhanced.
These findings highlight the PAD4-STAT1-PIAS1 axis as an immune restriction mechanism in macrophages and suggest that targeting this axis may have potential as a cancer immunotherapy strategy.
This test aimed to investigate the role of PAD4 in macrophages in tumor immune responses. The researchers conducted experiments using mouse models and different tumor cell lines.
1. Tumor Growth in Padi4-Deficient Mice
By inoculating MC38 cells (a murine colon adenocarcinoma cell line) subcutaneously into wild-type (Padi4+/+) and total-body Padi4 knockout (Padi4-/-) mice, it was observed that Padi4-/- mice developed smaller tumors compared to Padi4+/+ mice, as indicated by tumor volume and weight measurements.
2. Increased Levels of Tumor-Infiltrating Activated T Cells in Padi4-Deficient Mice
Fluorescence-activated cell sorting analysis revealed that Padi4-/- mice showed higher levels of interferon γ+ (IFNγ+) and interleukin-2+ (IL-2+) T cells in the tumor microenvironment (TME) compared to wild-type mice.
3. Specific Loss of PAD4 in Macrophages
To understand the role of PAD4 in T cells and macrophages, conditional knockout mouse strains were generated. Padi4fl/fl Cd4cre mice (with specific loss of PAD4 in T cells) did not show any difference in tumor volume compared to Padi4fl/fl mice. However, Padi4fl/fl LysMcre mice (with specific loss of PAD4 in macrophages) developed smaller tumors compared to wild-type Padi4fl/fl mice.
4. Enhanced T Cell Activation and Anti-Tumor Immunity in Padi4fl/fl LysMcre Mice
Padi4fl/fl LysMcre mice exhibited higher percentages of IFNγ+ and tumor necrosis factor alpha+ (TNFα+)IFNγ+ T cells in tumors and higher levels of IFNγ+ and TNFα+CD8+ T cells in tumor-draining lymph nodes compared to wild-type mice. Additionally, higher amounts of tumor-specific IFNγ+ T cells were detected in MC38 tumors in Padi4fl/fl LysMcre mice.
5. Effect on Tumor Metastasis
When Py8119 cells (a mouse breast cancer cell line) were inoculated subcutaneously, Padi4fl/fl LysMcre mice developed smaller tumors compared to wild-type mice. Furthermore, in an intravenous injection model using B16F10 cells (a murine melanoma cell line), Padi4fl/fl LysMcre mice had fewer lung tumor nodules compared to wild-type mice. Higher levels of IFNγ- and TNFα-expressing T cells were observed in the tumor microenvironment of Padi4fl/fl LysMcre mice.
Based on these findings, it was concluded that PAD4 in macrophages negatively regulates anti-tumor immunity, thereby promoting tumor progression.
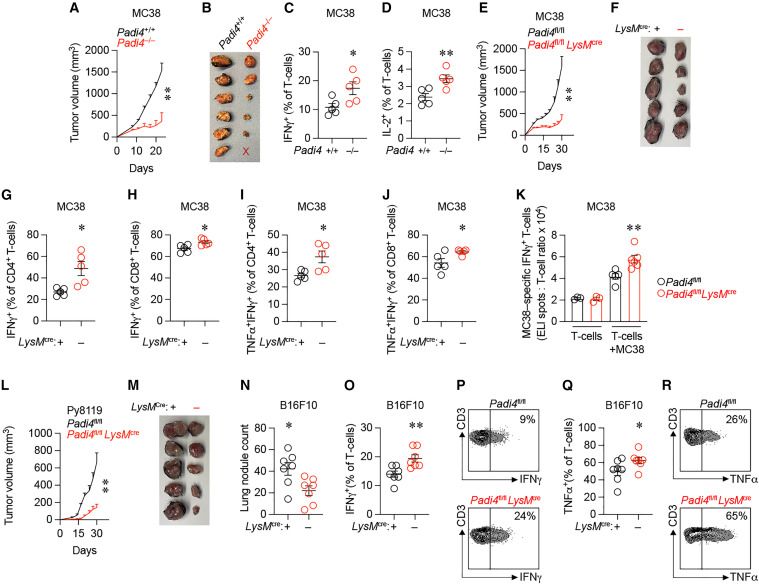
Case 2: Paunel-Görgülü A, Conforti A, Mierau N, Zierden M, Xiong X, Wahlers T. Peptidylarginine deiminase 4 deficiency in bone marrow cells prevents plaque progression without decreasing atherogenic inflammation in apolipoprotein E-knockout mice. Front Cardiovasc Med. 2022;9:1046273.
Plaque vulnerability and the risk of rupture in atherosclerosis depend on factors such as necrotic core size, collagen content, and inflammation. In this study, the researchers investigated the effect of peptidylarginine deiminase 4 (PAD4) deficiency on these factors in atherosclerotic lesions.
After 10 weeks of a high-fat diet (HFD), the size of the necrotic core increased significantly in both experimental groups compared to the size at 4 weeks. However, the necrotic core areas in mice with both ApoE gene deficiency and PAD4 deficiency (ApoE–/–/Pad4–/–-reconstituted mice) were significantly smaller than those in ApoE–/– control mice. This suggests that PAD4 deficiency is associated with reduced cell death in the necrotic core.
Furthermore, at the same time point, ApoE–/–/Pad4–/–-reconstituted mice showed a decreased abundance of α-SMA+ smooth muscle cells (SMCs) compared to control mice. α-SMA+ SMCs are known to contribute to the stabilization of atherosclerotic lesions. Additionally, Masson's Trichrome staining revealed reduced collagen content in the lesions of ApoE–/–/Pad4–/–-reconstituted mice. Collagen is an important component for plaque stability.
In contrast, the number of α-SMA+ cells and the collagen-positive area increased significantly in ApoE–/– control mice after 10 weeks of HFD compared to the earlier time point (4 weeks). However, this increase was not observed in ApoE–/–/Pad4–/–-reconstituted mice, indicating that PAD4 deficiency diminishes the abundance of collagen-producing SMCs.
Overall, these findings suggest that PAD4 deficiency reduces cell death in the necrotic core and leads to decreased abundance of collagen-producing SMCs, potentially affecting plaque stability in atherosclerosis.
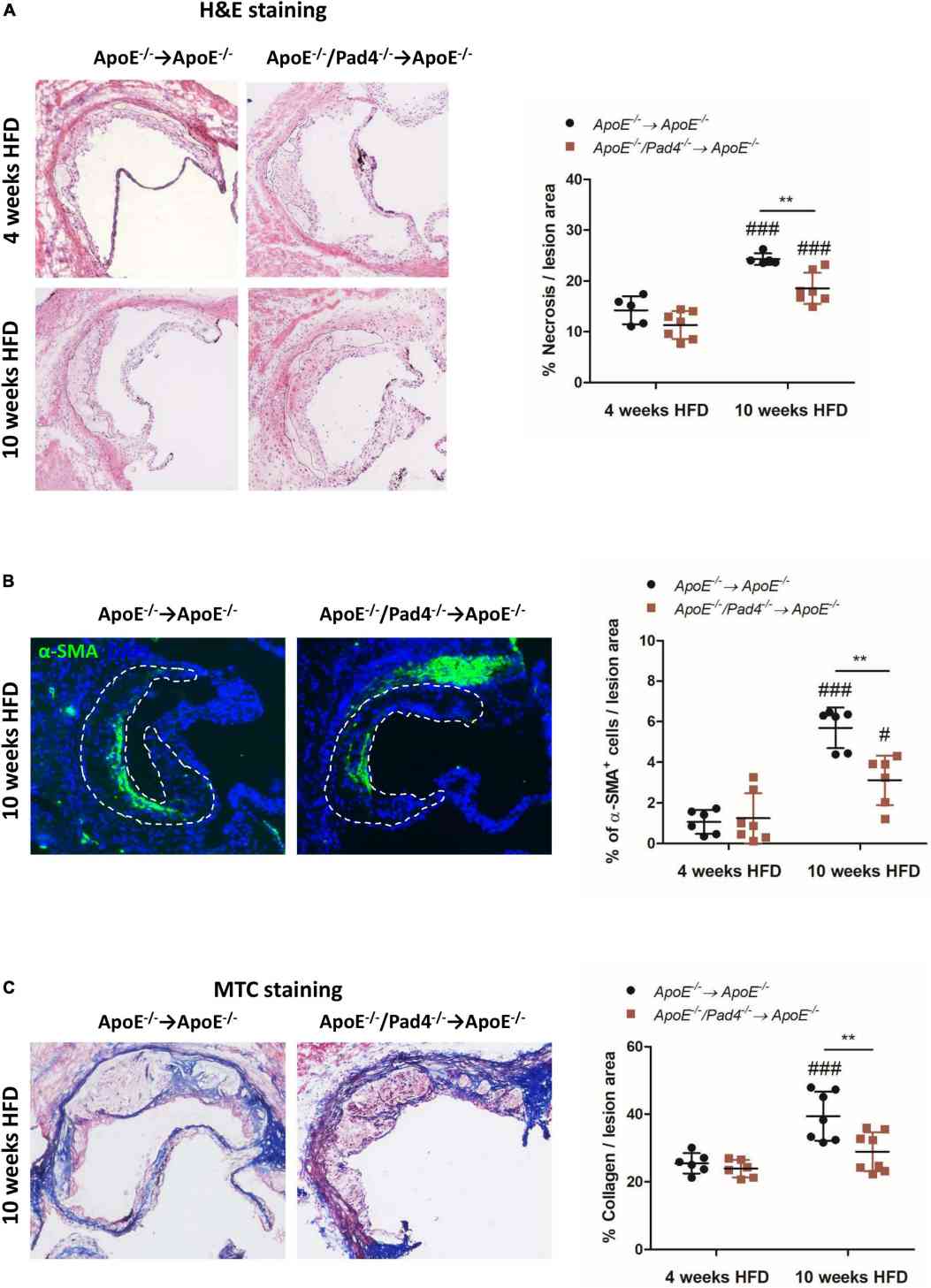
Related References
- Méchin MC, Takahara H, Simon M. Deimination and peptidylarginine deiminases in skin physiology and diseases. Int J Mol Sci. 2020;21(2):566.
- Zhu D, Zhang Y, Wang S. Histone citrullination: a new target for tumors. Mol Cancer. 2021;20(1):90.
- Zhu D, Lu Y, Wang Y, Wang Y. PAD4 and its inhibitors in cancer progression and prognosis. Pharmaceutics. 2022; 14(11):2414.